- 1Lydia Becker Institute of Immunology and Inflammation, Faculty of Biology, Medicine and Health, University of Manchester, Manchester, United Kingdom
- 2Centre for Respiratory Biology, University College London (UCL) Respiratory, Division of Medicine, University College London, London, United Kingdom
Trichuris muris, is the murine parasite and widely deployed model for the human whipworm Trichuris trichiura, a parasite that infects around 500 million people globally. Trichuriasis is a classical disease of poverty with a cycle of re-infection due to the continual exposure of humans, particularly children, to infective eggs, which contaminate the soil in endemic areas. Indeed, modelling studies of trichuriasis have demonstrated that the low efficacy rate of current anthelmintics combined with the high possibility of re-infection from the reservoir of infective eggs within the environment, mean that the elimination of morbidity due to trichuriasis is unlikely to occur. Despite the importance of the infective egg stage in the perpetuation of infections, understanding the biology of the Trichuris ova has been neglected for decades. Here we perform experiments to assess the impact of temperature on the embryonation process of T. muris eggs and describe in detail the stages of larval development within these eggs. In keeping with the early works performed in the early 1900s, we show that the embryonation of T. muris is accelerated by an elevation in temperature, up to 37°C above which eggs do not fully develop and become degenerate. We extend these data to provide a detailed description of T. muris egg development with clear images depicting the various stages of development. To the best of our knowledge we have, for the first time, described the presence of birefringent granules within egg-stage larvae, as well as providing a qualitative and quantitative description of a motile larval stage prior to quiescence within the egg. These experiments are the first step towards a better understanding of the basic biology which underlies the process of egg embryonation. With the threat of elevation in global temperatures, the accelerated embryonation rate we observe at higher temperatures may have important consequences for parasite transmission rates and prospective modelling studies. In addition, a deeper understanding of the Trichuris ova may allow the development of novel control strategies targeting the egg stage of Trichuris in the environment as an adjunct to MDA.
Introduction
Infections with the soil transmitted helminth Trichuris trichiura (T. trichiura) are highly prevalent with an estimated 465 million people infected worldwide in 2010, primarily in sub-saharan Africa (1). Infection with this nematode parasite causes the disease trichuriasis and occurs by the ingestion of embryonated eggs through contaminated soil or food. It is a classical disease of poverty, where a lack of education, poor sanitation and limited access to clean water results in increased infection transmission. In areas where trichuriasis is endemic, community prevalence of infection can be over 90%, with the highest parasite burdens occurring in children aged 2-15 years old (2). Within the infected population the infection intensity is high, with the majority of people harbouring low worm burdens but a small proportion of those infected (normally less than 10%) showing heavy worm burdens - over a hundred worms (2). Morbidity associated with trichuriasis is most commonly observed in those harbouring moderate or heavy infections, although this is hard to quantify due to the high prevalence of polyparasitism within infected populations (3). Observational studies on children with heavy infections have demonstrated that treatment with anthelmintics leads to significant extra gains in weight and height (4). It is possible that infections may also impact on cognitive function and educability although recent meta-analysis indicates that the evidence for an effect of anthelmintics, on both cognitive function and growth, is mixed (5–7).
Current tactics for controlling trichuriasis utilise a multi-pronged approach comprising anthelmintic treatment through mass drug administration (MDA) programs in combination with education about the parasite in endemic communities and increases in sanitation as part of the World Health Organisation (WHO) water, sanitation and hygiene campaign (WASH). The MDA programs are based on a preventative chemotherapy approach which target the main soil transmitted helminths (roundworm (Ascaris lumbricoides), whipworm (T. trichiura) and hookworms (Necator americanus and Ancylostoma duodenale)) and primarily treat pre-school and school-age children. This strategy is utilised as a more cost-effective approach than treating whole communities and is thought to reduce transmission within the whole community through targeting the primary parasite reservoir. However, whilst this strategy has been effective in targeting some of the other soil transmitted helminths e.g. Ascaris and hookworm, it has not been as successful in controlling trichuriasis especially in areas of Sub-Saharan Africa. This is largely due to the poor efficacy of current anthelmintic treatment against Trichuris. The current drugs recommended by the WHO for the treatment of STH infections are albendazole, mebendazole, levamisole and pyrantel pamoate. Most MDA programs currently use a single dose of albendazole or mebendazole repeated annually (8). However, a single dose of these benzimidazole compounds result in very low whipworm cure rates – 28% for albendazole and 36% for mebendazole (9). In recent years combination chemotherapy has been advocated by the WHO with albendazole-invermectin used on a large scale with cure rates estimated at approximately 60% however, this combination cannot be used in areas where Loa loa is prevalent due to fatal adverse effects. A more promising combination is albendazole plus the veterinary medicine oxantel pamoate with a cure rate of approximately 69% (10). However, oxantel pamoate is currently only approved for humans, as a monotherapy or in combination with pyrantel pamoate, in some countries of South America and Asia but not in all countries where trichuriasis is endemic (11).
Recently, the WHO has set new goals to achieve and maintain elimination of STH morbidity by 2030. However, modelling studies indicate that, as it stands, the low cure rates are insufficient to eliminate Trichuris morbidity or interrupt whipworm transmission, due to reinfection (12). Indeed, a large barrier to elimination of trichuriasis is the environmental contamination with parasite eggs. These eggs can persist in the soil for a long time and therefore, even following successful treatment of infected individuals, high reinfection rates occur. Whilst these problems can be minimised through water quality and sanitation improvements (latrine access, faecal waste management) the evidence from randomised controlled trials on WASH interventions is mixed. Indeed, in endemic communities there are significant difficulties in achieving and sustaining high levels of community coverage and behavioural change (13).
Despite the importance of the egg stage of the parasite in driving cycles of reinfection, and therefore morbidity, there is a sparsity of knowledge on the eggs and in particular the embryonation process. Indeed, the majority of the literature on egg embryonation across all Trichuris species is from the early 1900s. These studies reported the time the eggs take to embryonate and observed that embryonated eggs remain infective for at least 5 years (14). Brown, as well as studying the embryonation process in aqueous media, studied egg development in soil, which demonstrated an effect of humidity, temperature and soil type on the rate of embryonation of T. trichiura eggs. Recent studies looking at Trichuris muris (T. muris) eggs have concentrated on the cues required for the hatching process (15, 16) although there is still much to be determined (17). The one Trichuris species where the egg stage has been studied in more detail is Trichuris suis (T. suis), motivated by the therapeutic potential of T. suis ova (or TSO). These eggs have been proposed as a therapeutic candidate for autoimmune conditions like multiple sclerosis (18, 19) and inflammatory bowel disease (20) as well as conditions such as autism spectrum disorder (21) and thus, the generation of infective, embryonated eggs has been of interest to the field. Data from these experiments has demonstrated the importance of temperature in the embryonation process with an increase in temperate accelerating the embryonation process, although temperatures above 40°C cause the eggs to become degenerate (22).
In contrast to the relative wealth of knowledge on the embryonation of T. suis eggs, the primary paper which describes T. muris embryonation is a 1954 paper which elegantly describes the life cycle of T. muris from the unembryonated egg through to the adult stage of the parasite. In this experiment the author records that the first outlines of the larvae appear in 22-23 days when eggs are maintained at 25-26°C and that fully grown larvae are apparent by 30-31 days of incubation. The larvae within the embryonated eggs did not appear to moult and the fully grown larvae were described to be motile within the shells (23).
Given the importance of the egg stage of Trichuris in reinfection of individuals in endemic areas we set out to investigate the embryonation process in more detail. In keeping with the early works of Brown, Fahmy and Vejzagić we show that the embryonation of Trichuris eggs is accelerated at temperatures above 20°C until reaching temperatures when eggs degenerate. Notably we provide a more detailed description on T. muris egg development than provided before, a description of a motile larval stage within the egg shell and the presence of birefringent granules.
Methods
Animals
All experimental procedures were within the guidelines of the Animals (Scientific Procedures) Act, 1986. Severe combined immunodeficient (SCID) mice were bred in house at the University of Manchester and used at age 6-12 weeks. Mice were maintained at a temperature of 20-22°C in a 12h light, 12h dark lighting schedule, in sterile, individually ventilated cages with food and water ad lib.
Ethics Statement
All animal experiments were approved by the University of Manchester Animal Welfare and Ethical Review Board and performed under the regulation of the Home Office Scientific Procedures Act (1986). Experiments were performed under Home Office Licence P47AFBF29 and conform to ARRIVE guidelines.
T. muris Passage
The parasite was maintained as previously described (24, 25). Briefly, the parasite was passaged in susceptible SCID mice through infection with 150 infective T. muris eggs. At day 42 post infection the caecum and colon were removed, opened longitudinally, washed in pre-warmed RPMI (Merck, UK) supplemented with penicillin (Merck; 500U/mL) and streptomycin (Merck; 500µg/mL) (RPMI+5xP/S). Adult T. muris worms were gently removed using fine forceps and cultured in 6 well tissue culture plates to retrieve unembryonated eggs. Culture media was centrifuged at 720g for 15 min at room temperature and pelleted eggs were resuspended in 40mL deionised water, filtered through a 100µm nylon sieve and transferred to a cell culture flask. Flasks were kept in darkness, stored horizontally and eggs were monitored. After approximately 8 weeks eggs were fully embryonated and transferred for storage, horizontally at 4°C.
In Vivo Infection
For in vivo hatching assays, 40 eggs were counted under a dissecting microscope and given to a SCID mouse in 200 μL water. At day 15 post-infection the caecum and proximal colon were collected, blinded and stored at -20°C. For worm counts, frozen caecum and colon were defrosted in a petri dish containing water and cut open longitudinally. The gut contents were removed by swilling in the water and the gut tissue transferred to a fresh petri dish containing water. The gut mucosa was scraped off using curved forceps to remove epithelia and worms from the gut tissue. Both the gut contents and removed gut mucosa were then examined and T. muris worms counted using a dissecting microscope (Leica S8 APO).
Embryonation Assay
One hundred unembryonated eggs were placed in 1ml deionised water in eppendorf tubes in the dark at room temperature, 26°C, 30°C or 37°C either for 56 days or for shorter periods as described. Images were collected on an Olympus BX63 upright microscope using a 60x/1.42 PlanApo N (Oil) objective and captured and white-balanced using a DP80 camera (Olympus) in monochrome mode through CellSens Dimension v1.16 (Olympus). Images were then processed and analysed using the image analysis platform Fiji (18).
Matlab Analysis
An automated system was used to quantify larval movement within the egg. This was an adaptation of the previous published Paragon algorithm used for INVAPP measurements of motility of whole worms (26, 27). One hundred frame movies of eggs were recorded and motility determined by an algorithm based on thresholding pixel variance over time.
Results
The Rate of T. muris Egg Embryonation Is Altered by Temperature
The embryonation of T. muris eggs was investigated at several different temperatures to determine whether, akin to the published data in T. suis the rate of embryonation is impacted by the temperature of the eggs. In contrast to eggs which were left to embryonate at room temperature (approximately 19-21°C), the eggs which were maintained at 26°C and 30°C embryonated significantly faster with L1 larvae distinguishable within the eggs by week 2 in eggs maintained at 30°C and, in agreement with Fahmy (23), week 3 in eggs maintained at 26°C (Figure 1). In contrast, when eggs were maintained at 37°C the eggs failed to embryonate and appeared degenerate with the mass within the egg appearing to withdraw from the surrounding shell (Figure 1).
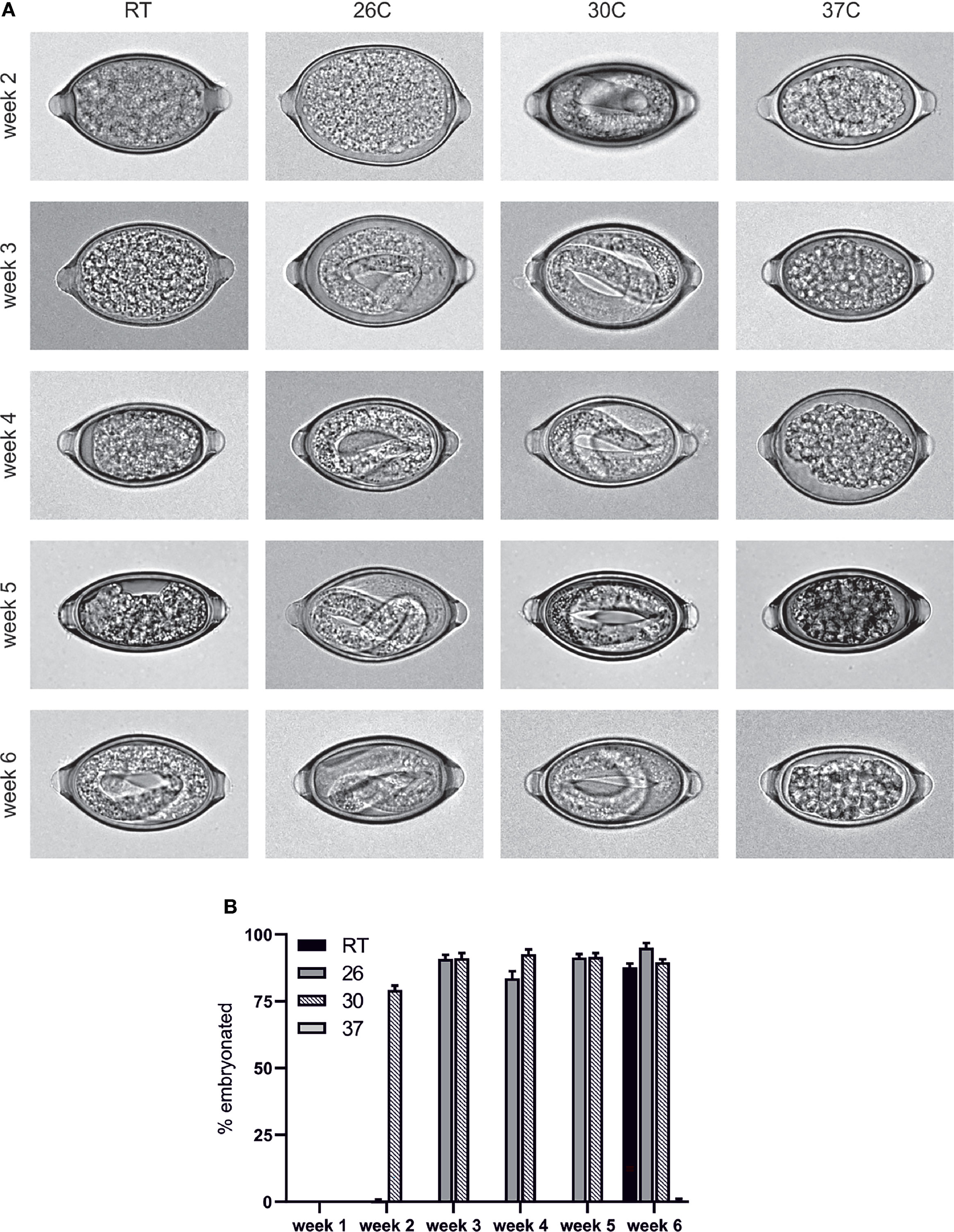
Figure 1 T. muris egg embryonation is a temperature dependent process. Unembryonated eggs were placed in deionised water at the specified temperature and embryonation determined at weeks 2, 3, 4, 5 and 6. (A) Representative pictures of eggs were imaged using an Olympus BX63 microscope with a 60x 1.42 PlanApo N (Oil) objective. (B) Embryonation rate was quantified by visual inspection of 100 eggs per sample (n=5).
The T. muris Egg Embryonation Process
In order to analyse the embryonation process in more detail we maintained the eggs at 26°C to provide a physiologically relevant but constant temperature to allow comparison between experimental repeats. Analysis of the eggs over multiple time points during the process of embryonation revealed T. muris eggs appear to follow a similar pattern to that described in T. suis eggs by Beer (28). Freshly deposited eggs which are collected from cultured worms are unsegmented and unfertilized and two nuclei-like areas are clearly evident within the cytoplasm which have been described to be male and female nuclei (Figure 2). As with embryonated eggs the unembryonated eggs are barrel shaped and contain a plug at each end. Measurements of these unembryonated eggs show they are approximately 60 µm in length and 30 µm wide. Within the first 1-2 days at 26°C a fusing of the two nuclei-like areas occurs followed by an unusual first division at approximately day 2 resulting in two blastomeres of unequal size. This asymmetric cleavage is not unique to T. muris, but is observed in several nematode egg embryonation processes (29). Subsequently, the larger blastomere then undergoes a further division resulting in a small blastula of 3 cells around day 3. This 3-cell mass then divides in 2 and subsequent cleavages occur until the blastula is formed of many small blastomeres (days 4-6). Prior to the generation of a larvae the mass of blastomeres appears to contract from one side of the eggs and then form into a cylindrical larva (day 7-14). Finally these cylindrical larvae develop into the infective L1 larvae with the characteristic oral spear of Trichuris clearly present by day 18 (see arrow Figure 2). Interestingly, we observed that once the L1 larvae are formed within the eggs there is a period of activity within the egg (days 21-28) prior to becoming quiescent by day 46.
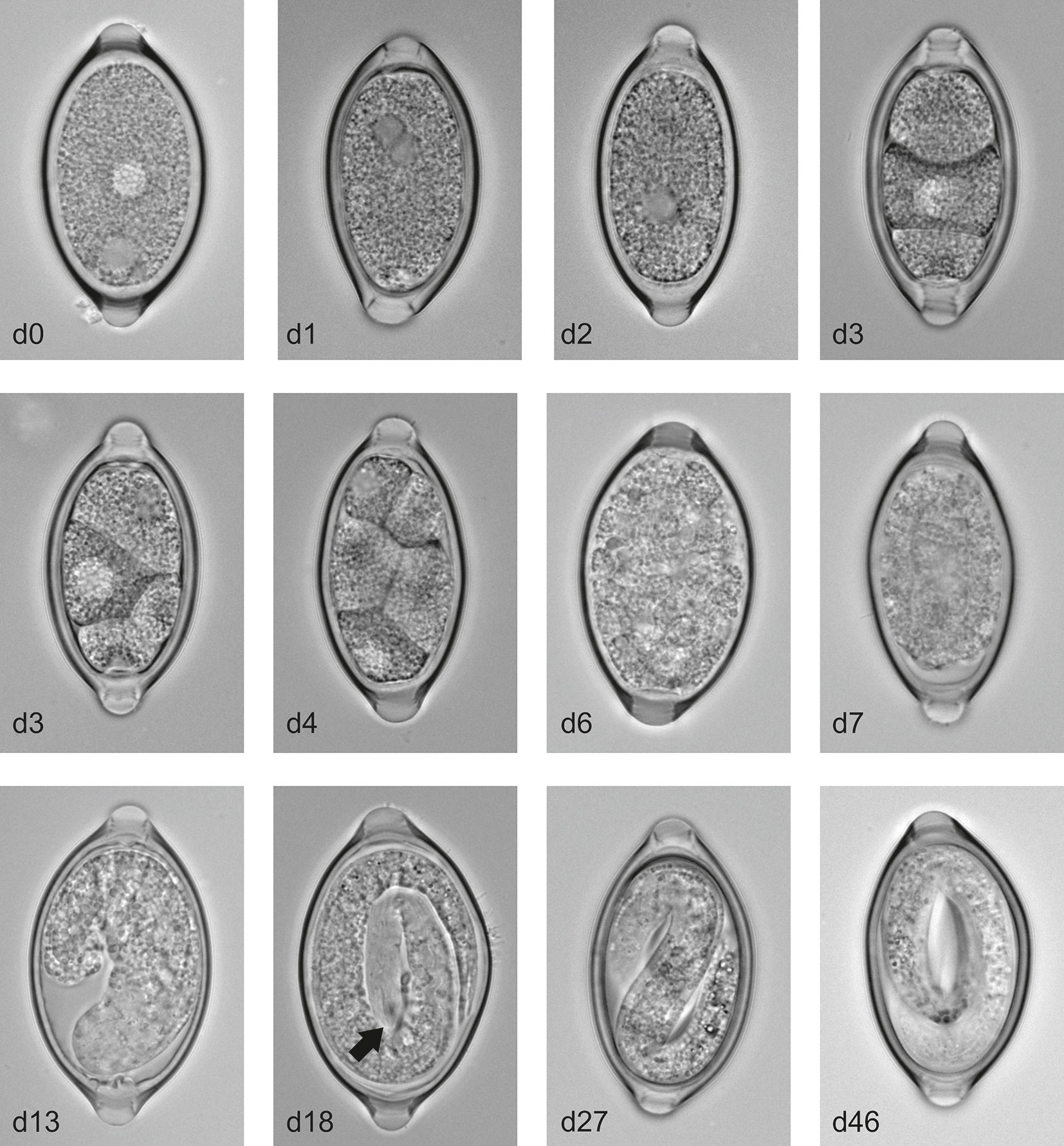
Figure 2 T. muris egg embryonation stages. Unembryonated eggs were placed in deionised water at 26°C and representative pictures of eggs were imaged at the specified time using an Olympus BX63 microscope with a 60x 1.42 PlanApo N (Oil) objective. Arrow indicates the characteristic oral spear of Trichuris.
The Motility of the T. muris Larvae Within the Egg
To further characterise the motile phase of the L1 larvae within the egg which, to our knowledge, has not be investigated in any depth, we characterised the length of the L1 larvae throughout this motile phase to determine if there is any change in size or moulting. However, there appears to be no significant changes in larval length (Figure 3A) and this is consistent with our inability to observe any moulting of the larvae within the egg. In addition, we generated time-lapse images of the eggs and applied a MatLab script to these images to determine the number of motile pixels above a threshold within the eggs, through the adaption of the previously published Paragon algorithm used for INVAPP measurements of motility (26, 27). This analysis demonstrated that newly formed larvae (around day 21-28) were most motile with motile granules evident within the larvae and the whole larva moving within the egg (Figures 3B, C and Supplementary Video 1). This movement then decreased with time and by day 46 movements were restricted to those of the motile granules within the egg (Figures 3B–E and Supplementary Video 2). These motile granules appeared to remain active indefinitely with active granules evident even in egg batches which have been stored at 4°C prior to bringing up to RT for imaging (data not shown). These motile granules are more visible under polarised light (Supplementary Video 3) and appear to be akin to the birefringent granules which have been described in C. elegans as gut granules within the intestinal lumen. To determine if these motile larvae are fully mature and infective we infected mice with these motile d28 larvae in comparison with fully mature eggs which had been embryonated at room temperature and then stored in the fridge (control eggs; as per our normal protocol). This demonstrated that these early newly-embryonated eggs do not display any defect in in vivo hatching (Figure 3F).
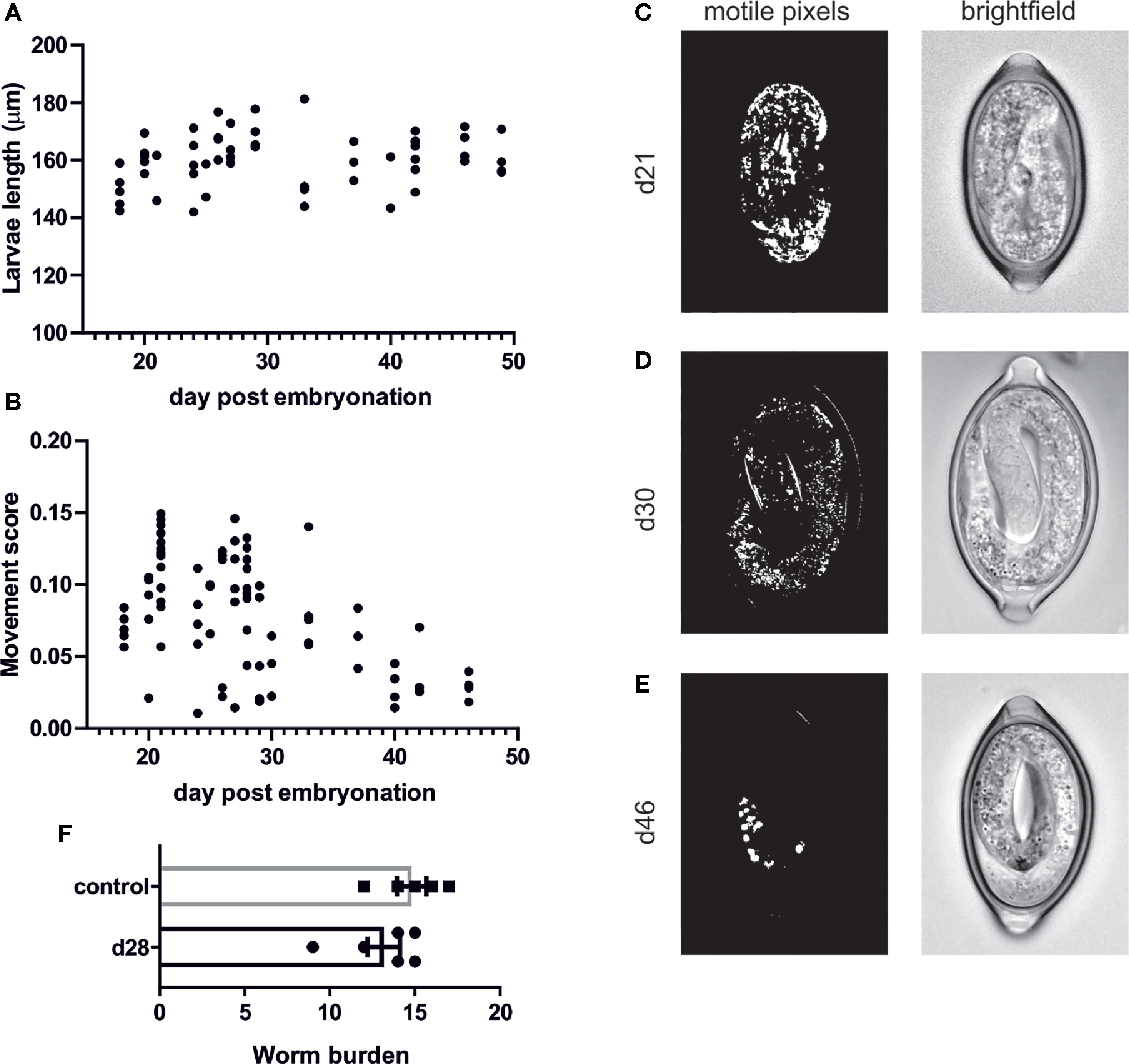
Figure 3 Quantification of T. muris L1 growth and movement during egg embryonation. Unembryonated eggs were placed in deionised water at 26°C and representative pictures of eggs were imaged at the specified time using an Olympus BX63 microscope with a 60x 1.42 PlanApo N (Oil) objective. (A) larval length was calculated on Z-stacked images on imageJ (B) movement score was calculated utilising MatLab analysis (C–E) Representative brightfield and matlab analysis pictures at selected time points (F) In vivo hatching assay to determine worm burden at d15 post infection.
Discussion
The embryonation of Trichuris spp. has been subject to very little research despite its importance in perpetuating the survival of the parasite. To the best of our knowledge we have, for the first time, described the presence of birefringent granules within the egg as well as providing a qualitative and quantitative description of the motile larval stage prior to quiescence within the egg. Although the purpose of the motile stage within the egg remains unknown, it does not appear to be required for larval maturation as the eggs are infective during this motile phase. Following quiescence of the mature larvae within the egg the birefringent granules appear to remain motile. Intestinal cell granules have been described in several nematodes including an initial report of them in adult Trichuris by Askanzy in 1896. This initial paper described reddish brown, weakly birefringent, ‘sphaerocrystals’ located in the anterior portion of the intestines of Trichuris nematodes. Despite their early description the function of these particles remains somewhat enigmatic, however some clarity about their role has emerged from experiments with the model nematode C. elegans. These experiments have shown the birefringent gut granules are lysosome-related organelles (30) and the characteristic birefringence and fluorescence is due to the presence of anthranilic acid (AA) glucosyl esters (31). The presence of anthranilic acid within the granules as well as their large size relative to ordinary lysosomes suggests they may function as a storage organelle (32). Indeed, it has been proposed that anthranilic acid may have antibiotic properties to help protect the larvae within the egg from pathogens (32). Furthermore, the granules have also been shown to contain zinc which is important in zinc tolerance as well as releasing zinc during dietary deficiency (33). Whether these proposed features of the granules in C. elegans are mirrored in the granules found in the T. muris egg is unknown. The observation that these granules remain motile, even in apparently quiescent larvae, may enable the analysis of egg transmissibility without the need to infect the host species. Indeed, through laboratory maintenance of T. muris eggs we know that a subset of embryonated eggs, which microscopically appear normal, do not subsequently hatch in vivo (unpublished). Whether this lack of infectivity associates with non-motile birefringent gut granules will be important to determine, enabling a deeper analysis of the impact of altering environmental conditions on not just embryonation but more importantly transmissibility.
In addition, our work shows for the first time the importance of temperature in the embryonation of T. muris. We demonstrate a clear temperature dependency of the rate of embryonation with an increase in temperature driving a significant increase up to 37°C beyond which, the eggs begin to degenerate. This is akin to the temperature dependent embryonation process which has been described in T. suis (22) and also fits with the observation of an optimum embryonation temperature of approximately 25-26°C by Fahmy (23). Importantly, in T. trichiura a clear relationship exists between the prevalence of infection and remotely-sensed mean land surface temperature (LST) with T. trichiura mostly prevalent in areas where the LST is between 25 and 33°C and almost eradicated in locations where the LST is over 38°C (34). These reported LST ranges fit well within the temperatures we have investigated with T. muris as a lack of prevalence of T. trichiura above 38°C fits well with our observations, and reports by others (22) of degeneration of eggs at 37°C. However, it is important to note that Trichuris eggs are not found only on the soil surface and have been reported to be detected at depths of up to 10cm (35) where temperatures may vary and the eggs are protected from direct sunlight. Indeed, helminth eggs have been shown to percolate deep into soil through both abiotic factors e.g. the leaching effects of rain (36) and biotic factors e.g. through association with earthworms (37).
Importantly, with the threat of increasing global temperatures and the rapid increase in the rate of embryonation over a relatively small temperature range, global warming may impact on parasite transmission rates. Indeed, it has been widely recognised that rising temperatures may influence the spread and transmission of parasitic diseases (38). If an increase in temperature from approximately 21°C (room temp) to 26°C in our experiments can halve the time required to generate fully embryonated eggs, than in countries like Madagascar where the parasite is already endemic these changes could increase the infection prevalence and the incidences of morbidity associated with the parasite. Indeed, the mean annual soil temperature in Madagascar is currently 20.2C (39) with an estimated increase in temperature predicted to be 1.8°C year−1 to 4.7°C year−1 for a doubling of CO2 (40). Should soil temperatures rise, our data suggests this would potentially result in an increased embryonation rate and therefore expansion of the parasite reservoir within the soil. Given that MDA programs are already struggling to have an impact on the prevalence this could be a serious stumbling block for the WHO goals to eliminate morbidity due to the prevalence of trichuriasis.
There is an urgent need to develop a deeper understanding of the processes which occur during embryonation. A better understanding of the basic biology which underlies the process of egg embryonation could help us access novel control strategies targeting the long-lived egg stage in the environment and thus reducing transmission of this soil transmitted helminth. Novel environmental control strategies deployed in known “hot spots” of open defaecation could offer a useful adjunct to MDA. Indeed some novel compounds have been shown to act against both embryonated and unembryonated eggs (41) and published Target Product Profiles for soil transmitted helminths list an effect against ova as a desired effect of novel anthelminitics.
Data Availability Statement
The original contributions presented in the study are included in the article/Supplementary Material. Further inquiries can be directed to the corresponding author.
Ethics Statement
The animal study was reviewed and approved by the University of Manchester Animal Welfare and Ethical Review Board.
Author Contributions
RF conceptualized the studies, designed experiments, performed research, analysed, and interpreted the data, and wrote the manuscript. FP wrote the analysis scripts, analysed and interpreted data and edited the manuscript. DS provided expertise in interpreting the data, contextualizing the studies, and editing the manuscript. KE conceptualized the studies, supported design of experiments, analysis, and interpretation of data, and wrote the manuscript. All authors contributed to the article and approved the submitted version.
Funding
RF and KE are supported by a MRC grant (MR/N022661/1) awarded to KE, DS and FP are supported by MRC grant (MR/N024842/1) and a UCL Therapeutic Innovation Networks Pilot Data Scheme award supported by funding from MRC UCL Confidence in Concept (CiC6) 2017 MC_PC_17180.
Conflict of Interest
The authors declare that the research was conducted in the absence of any commercial or financial relationships that could be construed as a potential conflict of interest.
Publisher’s Note
All claims expressed in this article are solely those of the authors and do not necessarily represent those of their affiliated organizations, or those of the publisher, the editors and the reviewers. Any product that may be evaluated in this article, or claim that may be made by its manufacturer, is not guaranteed or endorsed by the publisher.
Acknowledgments
Thanks goes to the University of Manchester bioimaging facilities. The Bioimaging Facility microscopes used in this study were purchased with grants from BBSRC, Wellcome and the University of Manchester Strategic Fund. Special thanks goes to Roger Meadows for his technical assistance.
Supplementary Material
The Supplementary Material for this article can be found online at: https://www.frontiersin.org/articles/10.3389/fitd.2021.790311/full#supplementary-material
Supplementary Video 1 | Representative brightfield video to demonstrate the whole larval movement observed at day 21 of embryonation (when maintained at 26°C).
Supplementary Video 2 | Representative brightfield video to demonstrate granule movement within the larvae at day 46 of embryonation (when maintained at 26°C).
Supplementary Video 3 | Representative video to demonstrate the birefringement granule movement under polarised light within the larvae at day 46 of embryonation (when maintained at 26°C).
References
1. Pullan RL, Smith JL, Jasrasaria R, Brooker SJ. Global Numbers of Infection and Disease Burden of Soil Transmitted Helminth Infections in 2010. Parasit Vectors (2014) 7:37. doi: 10.1186/1756-3305-7-37
2. Bundy D, Cooper ES, Thompson DE, Didier JM, Simmons I. Epidemiology and Population Dynamics of Ascaris Lumbricoides and Trichuris Trichiura Infection in the Same Community. Trans R Soc Trop Med Hyg (1987) 81:987–93. doi: 10.1016/0035-9203(87)90372-5
3. Pullan R, Brooker S. The Health Impact of Polyparasitism in Humans: Are We Under-Estimating the Burden of Parasitic Diseases? Parasitology (2008) 135:783–94. doi: 10.1017/S0031182008000346
4. Cooper ES, Duff EM, Howell S, Bundy DA. 'Catch-Up' Growth Velocities After Treatment for Trichuris Dysentery Syndrome. Trans R Soc Trop Med Hyg (1995) 89:653. doi: 10.1016/0035-9203(95)90430-1
5. Hall A, Hewitt G, Tuffrey V, De Silva N. A Review and Meta-Analysis of the Impact of Intestinal Worms on Child Growth and Nutrition. Maternal Child Nutr (2008) 4:118–236. doi: 10.1111/j.1740-8709.2007.00127.x
6. Pabalan N, Singian E, Tabangay L, Jarjanazi H, Boivin MJ, Ezeamama AE. Soil-Transmitted Helminth Infection, Loss of Education and Cognitive Impairment in School-Aged Children: A Systematic Review and Meta-Analysis. PloS Negl Trop Dis (2018) 12:e0005523. doi: 10.1371/journal.pntd.0005523
7. Taylor-Robinson DC, Maayan N, Donegan S, Chaplin M, Garner P. Public Health Deworming Programmes for Soil-Transmitted Helminths in Children Living in Endemic Areas. Cochrane Database Syst Rev (2019) 9:Cd000371. doi: 10.1002/14651858.CD000371.pub7
8. Who. Helminth Control in School-Age Children : A Guide for Managers of Control Programmes. Geneva: World Health Organization (2011).
9. Keiser J, Utzinger J. Efficacy of Current Drugs Against Soil-Transmitted Helminth Infections: Systematic Review and Meta-Analysis. JAMA (2008) 299:1937–48. doi: 10.1001/jama.299.16.1937
10. Speich B, Ali SM, Ame SM, Bogoch Ii, Alles R, Huwyler J, et al. Efficacy and Safety of Albendazole Plus Ivermectin, Albendazole Plus Mebendazole, Albendazole Plus Oxantel Pamoate, and Mebendazole Alone Against Trichuris Trichiura and Concomitant Soil-Transmitted Helminth Infections: A Four-Arm, Randomised Controlled Trial. Lancet Infect Dis (2015) 15:277–84. doi: 10.1016/S1473-3099(14)71050-3
11. Palmeirim MS, Specht S, Scandale I, Gander-Meisterernst I, Chabicovsky M, Keiser J. Preclinical and Clinical Characteristics of the Trichuricidal Drug Oxantel Pamoate and Clinical Development Plans: A Review. Drugs (2021) 81:907–21. doi: 10.1007/s40265-021-01505-1
12. Turner HC, Truscott JE, Bettis AA, Hollingsworth TD, Brooker SJ, Anderson RM. Analysis of the Population-Level Impact of Co-Administering Ivermectin With Albendazole or Mebendazole for the Control and Elimination of Trichuris Trichiura. Parasit Epidemiol Control (2016) 1:177–87. doi: 10.1016/j.parepi.2016.02.004
13. Vaz Nery S, Pickering AJ, Abate E, Asmare A, Barrett L, Benjamin-Chung J, et al. The Role of Water, Sanitation and Hygiene Interventions in Reducing Soil-Transmitted Helminths: Interpreting the Evidence and Identifying Next Steps. Parasit Vectors (2019) 12:273. doi: 10.1186/s13071-019-3532-6
14. Brown HW. Studies on the Rate of Development and Viability of the Eggs of Ascaris Lumbricoides and Trichuris Trichiura Under Field Conditions. J Parasitol (1927) 14:1–15. doi: 10.2307/3271397
15. Hayes KS, Bancroft AJ, Goldrick M, Portsmouth C, Roberts IS, Grencis RK. Exploitation of the Intestinal Microflora by the Parasitic Nematode Trichuris Muris. Science (2010) 328:1391–4. doi: 10.1126/science.1187703
16. Koyama K. Evidence for Bacteria-Independent Hatching of Trichuris Muris Eggs. Parasitol Res (2013) 112:1537–42. doi: 10.1007/s00436-013-3300-z
17. Mkandawire TT, Grencis RK, Berriman M, Duque-Correa MA. Hatching of Parasitic Nematode Eggs: A Crucial Step Determining Infection. Trends Parasitol (2021) 16:S1471-4922(21)00206-3. doi: 10.1016/j.pt.2021.08.008
18. Fleming J, Hernandez G, Hartman L, Maksimovic J, Nace S, Lawler B, et al. Safety and Efficacy of Helminth Treatment in Relapsing-Remitting Multiple Sclerosis: Results of the HINT 2 Clinical Trial. Mult Scler (2019) 25:81–91. doi: 10.1177/1352458517736377
19. Yordanova IA, Ebner F, Schulz AR, Steinfelder S, Rosche B, Bolze A, et al. The Worm-Specific Immune Response in Multiple Sclerosis Patients Receiving Controlled Trichuris Suis Ova Immunotherapy. Life (Basel) (2021) 11:101. doi: 10.3390/life11020101
20. Huang X, Zeng LR, Chen FS, Zhu JP, Zhu MH. Trichuris Suis Ova Therapy in Inflammatory Bowel Disease: A Meta-Analysis. Med (Baltimore) (2018) 97:e12087. doi: 10.1097/MD.0000000000012087
21. Hollander E, Uzunova G, Taylor BP, Noone R, Racine E, Doernberg E, et al. Randomized Crossover Feasibility Trial of Helminthic Trichuris Suis Ova Versus Placebo for Repetitive Behaviors in Adult Autism Spectrum Disorder. World J Biol Psychiatry (2020) 21:291–9. doi: 10.1080/15622975.2018.1523561
22. Vejzagić N, Kringel H, Bruun JM, Roepstorff A, Thamsborg SM, Grossi AB, et al. Temperature Dependent Embryonic Development of Trichuris Suis Eggs in a Medicinal Raw Material. Vet Parasitol (2016) 215:48–57. doi: 10.1016/j.vetpar.2015.10.031
23. Fahmy MA. An Investigation on the Life Cycle of Trichuris Muris. Parasitology (1954) 44:50–7. doi: 10.1017/S003118200001876X
24. Wakelin D. Acquired Immunity to Trichuris muris in the Albino Laboratory Mouse. Parasitology (1967) 57(3):515–24. doi: 10.1017/s0031182000072395
25. Forman R, Logunova L, Smith H, Wemyss K, Mair I, Boon L, et al. Trichuris muris Infection Drives Cell-Intrinsic IL4R Alpha Independent Colonic RELMα+ Macrophages. PLoS Pathog (2021) 17(7):e1009768. doi: 10.1371/journal.ppat.1009768
26. Buckingham SD, Partridge FA, Poulton BC, Miller BS, Mckendry RA, Lycett GJ, et al. Automated Phenotyping of Mosquito Larvae Enables High-Throughput Screening for Novel Larvicides and Offers Potential for Smartphone-Based Detection of Larval Insecticide Resistance. PloS Negl Trop Dis (2021) 15:e0008639. doi: 10.1371/journal.pntd.0008639
27. Partridge FA, Brown AE, Buckingham SD, Willis NJ, Wynne GM, Forman R, et al. An Automated High-Throughput System for Phenotypic Screening of Chemical Libraries on C. Elegans and Parasitic Nematodes. Int J Parasitol Drugs Drug Resist (2018) 8:8–21. doi: 10.1016/j.ijpddr.2017.11.004
28. Beer RJS. Morphological Descriptions of the Egg and Larval Stages of Trichuris Suis Schrank, 1788. Parasitology (1973) 67:263–78. doi: 10.1017/S0031182000046503
29. Schierenberg E. Embryological Variation During Nematode Development. WormBook (2006) 2:1–13. doi: 10.1895/wormbook.1.55.1
30. Hermann GJ, Schroeder LK, Hieb CA, Kershner AM, Rabbitts BM, Fonarev P, et al. Genetic Analysis of Lysosomal Trafficking in Caenorhabditis Elegans. Mol Biol Cell (2005) 16:3273–88. doi: 10.1091/mbc.e05-01-0060
31. Coburn C, Allman E, Mahanti P, Benedetto A, Cabreiro F, Pincus Z, et al. Anthranilate Fluorescence Marks a Calcium-Propagated Necrotic Wave That Promotes Organismal Death in C. Elegans. PloS Biol (2013) 11:e1001613. doi: 10.1371/journal.pbio.1001613
32. Gems D, Coburn C. The Mysterious Case of the C. Elegans Gut Granule: Death Fluorescence, Anthranilic Acid and the Kynurenine Pathway. Front Genet (2013) 4:151. doi: 10.3389/fgene.2013.00151
33. Roh HC, Collier S, Guthrie J, Robertson JD, Kornfeld K. Lysosome-Related Organelles in Intestinal Cells Are a Zinc Storage Site in C. Elegans. Cell Metab (2012) 15:88–99. doi: 10.1016/j.cmet.2011.12.003
34. Brooker S, Clements AC, Bundy DA. Global Epidemiology, Ecology and Control of Soil-Transmitted Helminth Infections. Adv Parasitol (2006) 62:221–61. doi: 10.1016/S0065-308X(05)62007-6
35. Paller VGV, Babia-Abion S. Soil-Transmitted Helminth (STH) Eggs Contaminating Soils in Selected Organic and Conventional Farms in the Philippines. Parasit Epidemiol Control (2019) 7:e00119. doi: 10.1016/j.parepi.2019.e00119
36. Storey GW, Phillips RA. The Survival of Parasite Eggs Throughout the Soil Profile. Parasitology (1985) 91( Pt 3):585–90. doi: 10.1017/S003118200006282X
37. Mizgajska H. The Role of Some Environmental Factors in the Contamination of Soil With Toxocara Spp. And Other Geohelminth Eggs. Parasitol Int (1997) 46:67–72. doi: 10.1016/S1383-5769(97)00011-1
38. Costello A, Abbas M, Allen A, Ball S, Bell S, Bellamy R, et al. Managing the Health Effects of Climate Change: Lancet and University College London Institute for Global Health Commission. Lancet (2009) 373:1693–733. doi: 10.1016/S0140-6736(09)60935-1
39. Ramifehiarivo N, Brossard M, Grinand C, Andriamananjara A, Razafimbelo T, Rasolohery A, et al. Mapping Soil Organic Carbon on a National Scale: Towards an Improved and Updated Map of Madagascar. Geoderma Regional (2017) 9:29–38. doi: 10.1016/j.geodrs.2016.12.002
40. Solomon S. Climate Change 2007 the Psysical Science Basis : Contribution of Working Group 1 to the Fourth Assessment Report of the Intergovernmental Panel on Climate Change. Cambridge: Cambridge University Press (2007).
Keywords: Trichuris, embryonation, egg, ova, neglected tropical disease (NTD)
Citation: Forman R, Partridge FA, Sattelle DB and Else KJ (2021) Un-‘Egg’-Plored: Characterisation of Embryonation in the Whipworm Model Organism Trichuris muris. Front. Trop. Dis 2:790311. doi: 10.3389/fitd.2021.790311
Received: 06 October 2021; Accepted: 28 October 2021;
Published: 18 November 2021.
Edited by:
Russ Russell Stothard, Liverpool School of Tropical Medicine, United KingdomReviewed by:
Philip John Cooper, St George’s, University of London, United KingdomCharles D. Mackenzie, Task Force for Global Health, United States
Copyright © 2021 Forman, Partridge, Sattelle and Else. This is an open-access article distributed under the terms of the Creative Commons Attribution License (CC BY). The use, distribution or reproduction in other forums is permitted, provided the original author(s) and the copyright owner(s) are credited and that the original publication in this journal is cited, in accordance with accepted academic practice. No use, distribution or reproduction is permitted which does not comply with these terms.
*Correspondence: Ruth Forman, ruth.forman@manchester.ac.uk