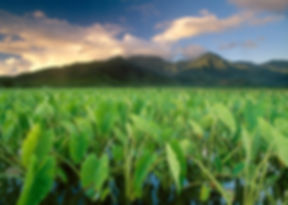
Riten Chand Gosai
MSc. - Tropical Plant Pathology (Honours)
RESEARCH PROJECT
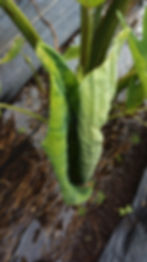
TaVCV -
New threat to Hawaiian Taro
Project | Phase 1 - Investigation into the Genetic Diversity of the Taro Vein Chlorosis Virus (TaVCV) in Hawaii
A taro leaf vein yellowing disease caused by the Taro vein chlorosis virus was previously found in eight (8) Pacific Island Countries and the Philippines. It was first reported in Hawaii in 2013. A consequent survey revealed that the disease is widepsread amongst the Hawaiian Islands. No further studies have been conducted thereafter. This research provides insights into the uncharted TaVCV disease in the Aloha State.
Phase 1:
TaVCV, first reported in Hawaii in 2013, has not been thoroughly studied hence the genetic variability of the virus in the state is unknown. "Evolutionary forces such as mutation, migration, genetic drift, selection and recombination change gene frequencies in populations and shape their genetic structure" (Peever et. al, 2000) . The goal here is to examine how different, genetically, the isolates are locally (that is, within the surveyed islands of Hawaii) versus a strain from Fiji (the only country in the Pacific to have the TaVCV genome fully sequenced).
Literature cited:
Peever, T.L., Zeigler, R.S., Dorrance, A.E., Correa-Victoria, F.J. and St. Martin, S. 2000. Pathogen Population Genetics and Breeding for Disease Resistance. APSnet Features. Online. doi: 10.1094/APSnetFeature-2000-0700

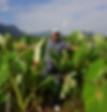
Me and my taro plants

Pippetting during different steps to sequence Hawaiian isolates of TaVCV

Marking bacterial colonies to be used for culturing in LB + Amp broth
To see more or discuss possible work >>>
Supplementary Reading - Overview of the Research
Introduction
Plants are infected by a wide variety of pathogenic micro-organisms such as fungi, nematodes, protozoa, bacteria and viruses (Agrios, 2005). Viruses are obligate parasites; intracellular, sub-microscopic particles that infect living organisms (Ellis, Boehm, & Feng, 2008). Viral infections result in many important plant diseases; responsible for decline in crop production and quality in all parts of the world. Viruses are also among the notable pathogens of taro, Colocasia esculenta.
Here in the Central and South Pacific where taro is an integral crop for the island countries, Dasheen mosaic virus (DsMV), Taro bacilliform virus (TaBV) or Taro badnavirus, Colocasia bobone disease virus (CBDV) and the Taro vein chlorosis virus (TaVCV) have been identified to cause leaf diseases on taro plants (Carmichael et al., 2008). Taro reovirus (TaRV) has also recently been reported, however, it’s etiology and symptomatology are not well understood (Revill et al., 2005b). DsMV and TaBV are relatively widespread throughout the Pacific whereas TaVCV and TaRV are restricted in their distribution. CBDV is confined to Papua New Guinea (PNG) and the Solomon Islands (Revill et al., 2005b; “Taro Research,” 2010).
From the above mentioned viral diseases, the taro vein chlorosis disease caused by TaVCV occurs in Fiji, the Federated States of Micronesia (FSM), New Caledonia, Palau, PNG, Solomon Islands, Tuvalu and Vanuatu (Davis & Ruabete, 2010; Harding, 2008;Revill et al., 2005b). Taro leaves infected by TaVCV show a characteristic feather-like chlorosis. This feathery yellowing broadens between adjacent veins as the leaf ages. The individual areas of chlorosis overtime converge into a network. During later stages of the disease cycle, the chlorotic areas may become necrotic near the leaf margins leading to a “tattered appearance” (Carmichael et al., 2008; Harding, n.d.). While the disease TaVCV produces is important on its own, a greater threat is the “alomae” disease. Severe alomae infections may result in 100% yield losses (Rodoni, Dale, & Harding, 1994). Taro plants contract this potentially lethal disease when two distinct viruses, TaBV and CBDV – another putative rhabdovirus, co-infect the same plant (Harding, 2008). As such, TaVCV is also considered a strong candidate capable of contributing to the onset of alomae (Carmichael et al., 2008; “Pests in Brief,” 2013).
The 2013 fourth quarterly release of the Pacific Pest Detector News officially published the first report of TaVCV in Hawaii and the United States. The disease was initially confirmed on the island of Molokai in March, 2013 following a study on taro plants that displayed leaves with veinal chlorosis and necrosis. Consequently, a survey of 35 sites on five of the Hawaiian Islands; namely Hawaii, Oahu, Maui, Kauai and Molokai revealed a 21.6% incidence of TaVCV in the samples collected. More importantly, positive samples were obtained from each of these five islands (Long at al. 2014). The researchers suggested the disease could not be a recent introduction in view of TaVCV’s widespread distribution around the state. No further studies on this virus have been conducted.
Discovery of TaVCV in Hawaii, alongside the already present DsMV and TaBV (Hu et al. 1995; Nelson, 2008; Melzer, Nelson, & Borth, 2013) has compounded the development of virus free germplasm for local and international distribution of this valuable staple crop. Further hindrance to germplasm sharing is the fact that TaVCV is understudied and the genetic diversity of TaVCV in Hawaii remains largely unknown. Owing to limited knowledge about the disease in Hawaii, just one detection technique is currently available – a simplex reverse transcriptase-polymerase chain reaction (RT-PCR) method. The primers for TaVCV RT-PCR were developed with sequence information from a few South Pacific island isolates (Revill et al. 2005a). Optimized RT-PCR assay with primers designed from sequences of Hawaiian isolates and serological detections tests are not available. Most importantly, TaVCV is not mechanically transmissible and the vector is unknown (Long et al., 2014; Revill et al., 2005a).
The goal of this proposed study is to examine the genetic diversity of TaVCV in Hawaii, develop improved molecular as well as a serological (enzyme linked immunosorbent assay – ELISA) detection technique and identify the vector which transmits TaVCV.
Justification
Taro has been an essential root-crop for Hawaiians and other Pacific Islanders since early times, hence embedded into the very fabric of Pacific culture and traditions (Caillon, Garcia, & Guarino, 2004). Taro or ‘kalo’ was revered as a supreme entity in ancient Hawaii (Jacobs, 2011) stemming out of the belief that human life emanated from the taro plants (Kelly, 2005). This notion is deep-seated and reflected in the Hawaiian description of a family unit, `ohana, derived from the word `oha, the suckers which grow from the main corm, makua (Cho, Yamakawa, & Hollyer, 2007). Hawaiian legends about creation tell of Haloa-naka, the first still-born child of the Gods from whose burial site a taro plant emerged. The next child, Haloa became the first human to live on the islands; he took care of the taro plant (his brother) and in return received sustenance. Taro is therefore sacred and idolized as an elder sibling to the Hawaiian people (Cho et al., 2007; “Taro,” 2014). In Palau, the taro patch is traditionally regarded as the provider of life exemplified in a native proverb as “the mother of our breath” (Bishop, 2013). Taro attracts similar socio-cultural significance in other Pacific Island Countries (PICs) where it is used in ceremonial gatherings and festivities (Caillon et al., 2004; Yalu, Singh, & Yadav, 2009; Liloqula, Saelea, & Levela, 1993; Pole, 1993; Primo, 1993; Taotua, 1993; Vilsoni, 1993). In this view, TaVCV or any taro disease poses considerable social and cultural peril to the Pacific Islands and its people.
In addition to being a dietary mainstay in PICs for generations, taro is a significant export commodity (McGregor et al., 2011). The Food and Agriculture Organization of the United Nations (FAO) ranked PNG, Fiji and Solomon Islands with-in the top 20 taro producers in the world at 5th, 14th and 19th spot respectively in 2013. PNG produced approximately 250,000 tonnes, Fiji 82,145 tonnes and Solomon Islands recorded 42,000 tonnes. Taro is also a noteworthy export produce for the Cook Islands as well (Caillon et al., 2004). Along with these large-scale producers, a recent Pacific Taro Market Access Study by McGregor et al. (2011) indicates Samoa, Tonga, American Samoa, Kiribati, Niue, Vanuatu, New Caledonia and Wallis and Futuna Islands are also prominent growers and consumers of taro. Locally, the state of Hawaii collectively produced a total of 10.7 million pounds (4,853,000 tonnes) of taro from 2011 – 2013 equivalent to USD7.014 million (“USDA Crop Production 2013 Summary,” 2014, “USDA Crop Values 2013 Summary,” 2014). Likewise, the Fijian taro industry is worth on average FJD20 million annually. These figures denote the high value of taro as one of the major domestic market as well as export crop. Unfortunately, rising pest and disease problems are encumbering taro production in Hawaii and the Pacific with harmful implications to food security, trade, social norms and cultural identity. Unique island geography means PICs are scattered away from each other in the vastness of the Pacific Ocean. This in fact is advantageous as plant pathogens including those of taro are then restricted in their distribution. For this reason, effective quarantine is critical to disease containment and management. However, the lack of user-friendly diagnostic tools impede quarantine measures as well as surveillance programs in these developing countries (Carmichael et al., 2008).
In addition to the above, internationally sharing germplasm collections of important crops such as taro is stifled if disease causing viruses are known to occur in an area, however, cannot be tested for and eliminated due to a lack of detection assays (Tuia et al., 2012). TaVCV is a definite candidate pertaining to taro. Germplasms may comprise of high yielding varieties or have cultivars with desired traits such as tolerance against climatic extremes, droughts and soil salinity. These collections essentially serve to protect and accumulate crops that may be vital in alleviating the effects of climate change and ensuring food security (“Taro Research,” 2010). Revill et al.(2005a) acknowledged that if countries are not able to offer germplasm exchange with disease free assurance, “access to agronomically elite varieties, hybrids and selected traditional cultivars are inhibited” and for these reasons sensitive diagnostic tests for TaVCV is a necessity. The pre-requisite to this is the knowledge of genomic information to enable the design of molecular and serological assays that will detect variant virus isolates.
There has only been one variability study of TaVCV in the Pacific utilizing 1.0 and 1.1 kb fragments of the polymerase (L) and nucleocapsid (N) genes respectively. Isolates from Fiji, FSM, New Caledonia, PNG, Solomon Islands and Vanuatu were studied. The L gene had a maximum variability of 27.4% at the nucleotide level and 11.3% at the deduced amino acid level. The N gene on the other hand had a maximum variation of 19.3% at nucleotide level and 6.3% at protein level (Revill et al. 2005a). However, the sequence diversity of TaVCV in Hawaii is still unknown and as a result such comparisons have not been made – within local isolates as well as against isolates from other PICs.
Vegetative propagation and supply of planting materials by farmers oblivious of infected plants may have contributed to the spread of TaVCV to different Hawaiian islands (Long et al., 2014). The unknown vector may then be carrying the virus even further. In view of all the aforementioned, this research aims to examine the genetic diversity of TaVCV in Hawaii, improve the molecular detection assay (RT-PCR) and develop a serological diagnostic technique (ELISA) for efficient detection of TaVCV as well as identify the vector which naturally spreads the virus.
Objectives
The objectives of this study are to:
-
Examine the genetic diversity of TaVCV in Hawaii
-
Improve molecular detection (RT-PCR) and develop a serological diagnostic test (ELISA) to detect variant TaVCV isolates in Hawaii
-
Determine the arthropod vector(s) which transmit the virus
Literature Review
Taro
1.0 Botany and Ecology
1.1 Classification
Taro belongs to the genus Colocasia in the Arum family, Araceae. In this family of monocotyledonous flowering plants, close to 100 genera and 1,500 species are recognized (Whitney, Bowers, & Takahashi, 1939). Thousands of years of cultivation in different regions of the world has led to the development of hundreds of varieties and agronomical cultivars. As a result, there is confusion and disagreement amongst botanists for systematic taxonomy of the genus Colocasia (Onwueme, 1999).
Hill (1939) provided a comprehensive description of the efforts of several botanists to classify taro: Linnaeus in 1753 described taro of two types; Arum colocasia and Arum esculentum. Schott in 1832 created the genus Colocasia and moved the above two species as Colocasia antiquorum and C. esculenta respectively. Schott also added a third species C. acris, earlier described as Calladium acre by Robert Brown in 1810. Kunth added a fourth species, C. nymphaeifolia in 1841 and Schott incorporated a fifth, C. fontanesii in 1854. In that same year, C. Koch and Sello reported a new species – C. euchlora which increased the number of species in the genus Colocasia to six.
Then, in 1856 Schott re-organized the classification and recognized only one species –C. antiquorum while the other five became ranked as varieties of this species. In 1879, Engler described two additional varieties; typica and illustris. Engler and Krause then added varieties aquatilis and globulifera in 1920. International Rules of Botanical Nomenclature otherwise known as the ‘Vienna Rules’ of 1906, later revised to International Code of Botanical Nomenclature – ‘Stockholm Code’ in 1952 rendered the changes made by Schott in 1856 as unlawful; according to one of the rules established by the consortium, an older species could not be reduced to a varietal rank under a new species published at a later date. Consequently, literature thereafter recognize C. esculenta as the main polymorphic species of the genus Colocasia and antiquorum as a variety (Hill, 1939). Commonly cultivated taro belong to two botanical varieties; Colocasia esculenta (L.) Schott var. esculenta and Colocasia esculenta (L.) Schott var. antiquorum (Purseglove, 1973).
C. esculenta var. esculenta has edible underground stems called corms and bud-like smaller structures called cormels. Above ground, the leaves and petioles of certain cultivars are also consumed (Alercia, 2013). In comparison, C. esculenta var. antiquorum has a small globular central corm, with several relatively large cormels arising from this inner corm. C. esculenta var. esculenta is agronomically referred to as the dasheen type of taro while C. esculenta var. antiquorum is called the eddoe type. The majority of taro cultivated in the Asia-Pacific region is the preferred dasheen type (Onwueme, 1999).
Due to the widespread distribution of taro, there are many cultivars grown throughout the world. These cultivars may be differentiated on the basis of physiological characteristics such as shape and size of the corm or shape, size, color and texture of the leaves and petioles. Additionally, agronomic or culinary predilection may also be used to distinguish cultivars (Onwueme, 1999). Historical accounts ascertain that Hawaiian people grew approximately 300 cultivars of taro; both wetland and dryland types even before first contact with Europeans in 1778. These cultivars were segregated on the basis of leaf color variation and their ability to grow in specific conditions and locations (Cho et al., 2007; Jacobs, 2011). Whitney et al. (1939) making reference to MacCaughey and Emerson (1914) stated that approximately half of these 300 varieties are the same of different names.
1.2 Origin and Distribution
Taro is thought to have originated in South Central Asia, most likely in India or the Malay Peninsula (Hill, 1939; Whitney et al., 1939). Conversely, a karyotypic study points at North-eastern India as the center of origin of taro (Kuruvilla & Singh, 1981). From its source, taro spread eastwards to the rest of South East Asia, China, Japan and the Pacific Islands with the early seafaring voyagers. At the same time, it was taken westward to Egypt and the eastern Mediterranean; and then southward and westward to East and West Africa and likely introduced to the Caribbean and the Americas from there (Lee, 1999; Matthews, 1995, 2004; Onwueme, 1999; Plucknett, 1983).
Interestingly, an isozyme variation study of taro cultivars from Asia and Oceania identified a higher level of genetic diversity in Asian taro while pointing to a distinctive gene pool in the Pacific. Indonesian cultivars had the highest genetic variation and results indicated Pacific cultivars may have originated from a narrow base within this gene-pool (Lebot & Aradhya, 1991). Noyer et al. (2003) observed similar differentiation between Southeast Asian and Melanesian taro using a simple sequence repeat (SSR) marker technique thereby confirming the isozyme results. These two observations were further supported by Kreike, Van Eck, & Lebot (2004) with the use of amplified fragment length polymorphism (AFLP)-PCR. Phylogenetic analysis from their research virtually conclusively separated 255 taro accessions into Asian and Pacific gene pools. These results elucidate possibly two separate domestication processes and Deo et al. (2009) suggested that taro can therefore be recognized as a native plant of the Pacific as well.
Polynesian migration into the Hawaiian Islands is regarded as the primary source of introduction of C. esculenta into Hawaii (Greenwell, 1947). Off the hundreds of taro cultivars developed by Hawaiians prior to European arrival, 84 were documented in 1939 by Whitney et al.; information on which is accessible at this University of Hawaii at Manoa (UHM) - College of Tropical Agriculture and Human Resources (CTAHR) online catalogue: http://www.ctahr.hawaii.edu/site/Taro.aspx. The bulk of the taro produced in Hawaii now is dominated by the varieties ‘Maui lehua’ which has extensively overtaken the once-preferred ‘Lehua Maoli’; then ‘Moi’ and ‘Bun Long’(Cho et al., 2007). The widespread cultivation of only a few selected varieties has been attributed to large scale commercial farming and research work that promote certain varieties over another. The notion exists that taro in Hawaii has been transformed from a commodity once sacrosanct and grown widely throughout the state to merely a crop mono-cultured in non-traditional ways (Jacobs, 2011).
1.3 Morphology and Anatomy
Taro, which has been referred to as the "potato of the tropics," is a herbaceous perennial plant that grows 1 – 2 m tall and covers a similar area with the spread of its canopy. A detailed taxonomic description was documented by Whitney et al. (1939):
“Extremely variable, succulent, glabrous herb, 4 to 18 dm. tall. Stem a subterranean corm with scaly outer bark and thin, usually highly colored cortex, single or branching from the apex, with conspicuous leaf-scar rings, producing cormels (aha) or rhizomes as offshoots. Petioles 4 to 18 dm. long, erect or spreading, sheathing at base with sinus to about midway, uniformly light or dark green to variously highly colored, striped, or flecked. Blades 25 to 85 cm. long, 20 to 60 cm. wide, usually peltate, ovate to more or less sagittate, the apex acuminate, a dark-colored spot known as pika on the upper surface at the point of junction with petiole. Inflorescences 2 to 5 together in the leaf axils, the peduncles 15 to 50 cm. long, each spadix enclosed within a spathe. Spathes oblong-lanceolate, divided by a transverse constriction into two unequal parts, the lower part 3 to 5 cm. long, loosely or tightly convolute, more or less fleshy, tubular, the upper part 15 to 35 cm. long, usually tightly but sometimes loosely convolute, lanceolate. Spadix 6 to 14 cm. long, with female flowers at the base, consisting of a few obovoid or ellipsoid ovaries 0.5 to 1.5 mm. in diameter, the stigma sessile, capitate; constricted above the female flowers and beset over a length of 2 to 5 cm. with light yellow sterile flowers; above the sterile flowers and over a length of 2 to 4 cm. beset with male f1owers,consisting of 2 to 6 sessile anthers which are fused into an obconical synandrium; with yellow constricted, obtuse or acute sterile appendage at apex. Fruit a berry, 3 to 5 mm. across, ellipsoid. Seed 1.2 to 1.5 mm. long, 0.7 to 1 mm. wide, hard, ovoid.”
The underground consumable central corm represents the main stem structure of the plant. In the dasheen type of taro, which is the subject of this study, the corm is cylindrical and large; shaped like a top with rough ridges, lumps and fibrous gangling roots. The corms can grow up to 30 cm long and 15 cm in diameter, generally weighing around 1-2 pounds, but can weigh up to eight pounds (Moore & Lawrence, 2003; Onwueme, 1999).
The taro corm and almost all other parts of the plant contain idioblasts (cells with raphides or bundles of calcium oxalate crystals). These compounds are responsible for the acridity or itchiness of taro and for this reason it is not recommended to consume any part of the plant uncooked. The density and woodiness of the corm increases with age. The skin covering the corm is brown with white or pink flesh. Certain varieties of C. esculenta are known to produce smaller tubers or cormels which grow off the sides of the main corm. The cormels are also edible. Although rare, some taro plants shoot off runners as well. These grow horizontally along the soil surface for a short distance, rooting down at intervals to give rise to new standing plants (Moore & Lawrence, 2003; Onwueme, 1999).
2.0 Ethnobotany of Taro
2.1 Food Security, Nutritional and Economic Importance of Taro
Taro is one of the major staple root-crops in all PICs, as such integral to the region’s food security, nutrition and economy. Historically, taro topped the ranks as the most important crop grown throughout the Hawaiian Islands (Moore & Lawrence, 2003). Although it no longer holds that status in comparison with crops such as nuts, seed corn and coffee in present-day Hawaii (Gomes, 2012), taro is still consumed in considerably large quantities throughout the PICs and in a variety of ways.
Taro corm, the main edible part of the plant is boiled, baked or fried and consumed with an array of vegetable and meat preparations. In what is a favorite Pacific cuisine, taro along with other staple crops and meat is cooked on hot stones in dug-out earth ovens, known as imu in Hawaii, umu in Samoa and lovo in Fiji (Deo et al., 2009). This is a common preparation method employed during traditional ceremonies, festivities and celebrations. Young taro leaves cooked with coconut cream, canned meat or tuna, called palusami is another delicacy – a rich side dish prepared in the imu, umu or lovo. Fiji-Indians make curry from both the corm and the leaves of the taro plant. The inflorescence is also a delicacy in some Asian and Pacific food cultures (Rao et al. 2010).
In Hawaii, taro was “the staff of life” for early settlers (Wang & Higa, 1983; Whitney et al., 1939) made into the symbolic dish poi (Potgieter, 1940) – a fermented or unfermented paste achieved by mashing cooked corm with water to a desired consistency (Lee, 1999). Another authentic Hawaiian dish, lu’au, has been passed down from generations; made by cooking young tops and leaves with coconut cream and poi, the sour paste made from boiled taro (Uchida et al., 2008). Commercially, taro has long been processed into flour and beverages (Payne, Ley, & Akau, 1941) and more recently into taro chips (Hollyer, Paull, & Huang, 2000) in several PICs. Taro flour, to some extent, is also utilized in the pastry and baking industry (Ammar, Hegazy, & Bedeir, 2009). Even then, the intensity of taro cultivation has dropped over the years and taro is now regarded as a highly nutritious yet under-utilized commodity (Alercia, 2013); nonetheless certainly one that is vital for the region’s food security.
Nutritionally, the taro corm is rich in complex carbohydrates and is a primary source of starch, the dietary contribution of which is similar to potatoes. Taro starch has a digestibility value of 98.8 percent owing to the minute size of its granules which are ten times smaller than that of potato starch (Jane et al., 1992). For this reason, taro flour is an ideal constituent for the production of infant formulae and is also an important ingredient of canned baby foods. Additionally, as a substitute, taro is beneficial for persons with digestive problems and allergies to cereals; and for children sensitive to milk (Lee, 1999; Moore & Lawrence, 2003). Taro leaves are rich in protein while the corm has very little fat and contains dietary fiber. The leaves and corm contain several essential vitamins such as E, C (ascorbic acid), B1 (thiamin), B2 (riboflavin), B6, B9 (folic acid) and macro and micro minerals; namely magnesium, phosphorous, copper, potassium, calcium, iron and manganese (Alcantara, Hurtada, & Dizon, 2013; Alercia, 2013; Payne et al., 1941; Potgieter, 1940; Temesgen & Retta, 2015). Alternatively, researchers have also recommended the use of taro corms, leaves and petioles as important ingredients in animal feed (Adejumo, Babalola, & Alabi, 2013; Moore & Lawrence, 2003).
Furthermore, the taro plant has a variety of traditional and herbal medicinal purposes. In Africa, fresh leaves are used for blood clotting, soothing wounds, sores and boils while in Indonesian juice from the stalk is employed to neutralize snake poison and root extract is used to treat rheumatism and acne (Abbas, 2011). In the Philippines, leaves and corms were prepared in special ways to help ease pain during childbirth. Some tribes used early morning dew on the leaves as eyewash, sitting on taro leaves helped women with dysmenorrhea while the styptic petiole juice was used to arrest arterial hemorrhage. Taro plants also provided remedy for ear aches and were used as laxative to treat hemorrhoids or as antidotes for stings. Mixing tuber ash with honey helped cure mouth apthae. In Hawaii, people suffering from fever were made to drink raw taro leaf juice mixed with sugar for relief. Taro provided remedies for many other illnesses including constipation and tuberculosis (Moore & Lawrence, 2003). Poi has been shown to benefit infants with food allergies and treat “failure-to-thrive” conditions. It also has use as a probiotic (Brown & Valiere, 2004).
Taro is one of the few unique commodities of which both the above and below-ground parts are consumed. It is therefore an important domestic market fresh crop in almost all PICs (McGregor et al., 2011). Revamped production in recent years has also enabled a few countries in the region, namely Fiji, Samoa, Tonga and Vanuatu to open export markets for their taro (Sami, 2011). FAO assessments show that between 2005 and 2009, Pacific countries produced on average a combined 430,000 tonnes of taro. During that same period, 10,000 to 13,000 tonnes of taro were exported annually to countries such as Australia, New Zealand, USA and Japan. Fiji, the highest exporter accounted for almost 95 percent of total exports valued around FJD20 million per annum (McGregor et al., 2011). The export figures seem low compared to the total production figures because PNG, the largest grower of taro in the Pacific (representing over half of the total production) favors domestic sale. Additionally, shipping costs to trading partners are high meaning it cannot offer taro at competitive prices like Fiji (McGregor et al., 2011). Locally, Hawaii produced 3.1 million pounds (1,406 tonnes) of taro in 2013 worth over USD1.9 million (“USDA Crop Production 2013 Summary,” 2014, “USDA Crop Values 2013 Summary,” 2014).
2.2 Socio-cultural Significance of Taro to Hawaii and the Pacific Islands
Taro has its roots entrenched deep into Pacific history and has impacted daily life, more so in the Polynesian countries like no other crop has. For native Hawaiians, daily routine revolved around the lo’i (flooded taro patches) and taro farming formed the basis of early civilization (Greenwell, 1947). Over time taro has attained significant socio-cultural importance not only in Hawaii but other PICs as well, some of which are discussed below.
Taro cultivation was not merely an activity for ensuring food availability in early Hawaii; it had strong attachment to beliefs about creation. This is portrayed in the Hawaiian creation chant – Kumulipo. The chant is a narrative of how taro is the plant from which Hawaiians have descended. The Kumulipo conveys the legend of Papa, Earth Mother and Wakea, Sky Father who gave birth to Ho’ohokukalani, the most charming and endearing woman of all times. Ho’ohokukalani’s first child, Haloa-naka, was still born hence entombed in the earth. From the site where Haloa-naka was buried germinated the Kalo or taro plant. This plant provided sustenance to the second born; Haloa-naka’s younger brother, Haloa. Because taro is vegetatively propagated, Haloa-naka lives on. In this way, the taro plant is superior and more sacred than man himself; it is an elder sibling deserving care and respect. It is the younger son Haloa from whom Hawaiians delineate their ancestry (Cho et al., 2007; Jacobs, 2011; Uchida et al., 2008).
In olden days Hawaii, prayers were offered to the Gods to seek blessings every time a new activity commenced during the farming cycle. These occurred right from land preparation to harvesting the kalo and consumption. The venerations dignified the act of farming and provided significance to the work done to produce taro. Intertwined with social-norms, the agricultural system of the past was not designed for profit making. It rather focused on feeding communities, maintaining religion, practicing tradition and upholding customary obligations (Uchida et al., 2008). This symbolism and spiritual connection conferred an ambience of mythology upon the taro plant and its history in Hawaii (“Taro,” 2014). Although the industry has been commercialized, this representation continues to exist. Art in various forms centered around the theme of kalo are visible throughout the state, a testimony to how taro continues to be an influence in Hawaii’s food and agricultural landscape (Deo et al., 2009).
An enthralling evidence to this emotional attachment to taro is the case of patenting efforts by UHM – CTAHR’s plant pathologist Eduardo Trujillo who introduced three improved taro varieties in 2002 after an extensive breeding program started in the early 1990s (“CTAHR and Taro,” 2009). These varieties were resistant to taro leaf blight (TLB) and also possessed desired traits from the local cultivar Maui Lehua (Trujillo, Menezes, Cavaletto, Shimabuku, &Fukuda, 2002). Maui Lehua is one of the cultivars in the royal Lehua family of Hawaiian cultivars. The displeasure of the local community in relation to the indigenous and cultural sentiments attached with taro versus ownership of the new varieties by the university resulted in protests in 2006. Consequently, UHM renounced its patent rights and made the varieties available publically (“CTAHR and Taro,” 2009). Kalo was designated the state plant of Hawaii in 2007. Following this announcement, the Hawaii County Council issued a ban on genetically modified taro (and coffee) on Big Island in 2008 by passing a bill which made it illegal to “test, propagate, cultivate, raise, plant, grow, introduce or release genetically engineered taro and coffee” in the county. Citing taro as a sacred plant, the Maui County Council followed with a similar suit in 2009 (Jacobs, 2011).
Elsewhere in the Pacific, Palauans also have a strong affiliation with the taro plant and believe in an age-old proverb that states “the taro patch is the mother of our breath”. Taro is not only a source of food and income in Palau, but a token of exchange and gratitude during cultural customs and family events such as birth ceremonies and funerals (Bishop, 2013). Similarly, taro is superior over all other root crops and the preferred commodity for royalty, gift-giving, traditional feasting and the fulfillment of social obligations in Fiji (Vilsoni, 1993), Samoa (Taotua, 1993), Tonga (Pole, 1993), the Solomon Islands (Liloqula et al., 1993), Micronesia (Primo, 1993) and many other countries in the South Pacific (Sivan & Liyanage, 1993). Cultivated in the region for centuries, taro also has mentions in the oral folklore, myths and legends of many Pacific cultures (Deo et al., 2009). Taro inherently is a symbol of cultural identification synonymous to Pacific lifestyle and the people, wherever they live in the world (Onwueme, 1999). The socio-cultural prominence of taro across the PICs is unmatched by any other root crop or agricultural commodity and this prestige will continue to persist having endured the test of time after first being domesticated in the region thousands of years ago.
3.0 Known Pests and Diseases of Taro
3.1 Pests
Taro beetles, Papuana spp. (Coleoptera: family Scarabaeidae) are perhaps the most important pests of taro in the South Pacific region. These shiny black scarab beetles, about 15-25 mm in size at the adult stage have been reported to cause extensive damage to the corm in PNG, Solomon Islands, Vanuatu, Kiribati and Fiji. Other aroids in the taro family are also vulnerable to taro beetle attack (Aloalii et al. 1993). Burrowing action into the corms creates tunnels and exposes the flesh, thereby providing entry points for other pathogens. This secondary rotting is a major contributor to corm spoilage in the field as well as in post-harvest storage (Lal et al., 2008). Taro beetles have not been reported in Hawaii, however, globalized trading routes and rapid people movement do present the risk of accidental introduction. Quarantine efforts are therefore paramount in keeping this pest from arriving into the state.
Conversely, a pest of concern in Hawaii is the taro root aphid, Patchiella reaumuri. It was first discovered on Big Island (Hawaii) in 1971 then on Oahu in 1995. The taro root aphid is particularly destructive for dryland production but has not been problematic in wetland taro. It is thought to be host specific, presumably feeding only on taro and a few related plants within the family. While the aphids are yellow-gray in color, their colonies are usually covered with a mass of fine, white, cottony, waxy substance. Winged sexual types of the taro root aphid have not been detected in Hawaii; reproduction occurs without male fertilization. Heavy losses of 75-100 percent in certain varieties have been reported as a result of taro root aphid infestation. Damage is escalated by drought conditions and is severe on young plants (Sato et al. 1997).
Other pests of concern include the taro planthoppers (Tarophagus spp), heavy infestation by which may result in the wilting and death of plants; hawk-moth (Hippotion celerio), the larvae of which may heavily feed on the leaves causing defoliation; and the armyworm caterpillars (Spodoptera litura) that also consume the leaves (Carmichael et al., 2008; Deo et al., 2009). Spiraling whitefly, Aleurodicus dispersus, the aphid, Aphis gossypii and mealybugs (Family Pseudococcidae) are also common pests. Widely distributed across the Pacific, their piercing and sucking action can also cause wilting and death in heavy infestations. Exudated honeydew promotes the growth of sooty moulds that impede photosynthesis. Tobacco whitefly, Bemesia tabaci, has also been noted for similar, but less common damage. Additionally, spider mites (Tetranychus spp.) cause whitish-yellow speckling and premature death of leaves (Carmichael et al., 2008) while mealybugs have long been linked to TaBV transmission (Gollifer et al., 1977; Macanawai et al., 2005).
Finally, snails round up the list of pests for taro. The giant African snail (GAS), Lissachatina fulica, found in many PICs has been sighted feeding on the leaves. Carmichael et al. (2008) report that the level of damage is directly related to the population; in areas where numbers are low, GAS feed on alternative, more preferred plants such as garden cabbage. Apple snails, Pomacea canaliculata present on all islands except Molokai and Lanai and Pila conica present only on Molokai are major threats to Hawaiian taro. They were introduced from South America for the Hawaiian aquaculture industry, however, escaped and began appearing in taro patches in 1983-1984. These snails are rapid invaders and infest in large numbers, hence damage significant taro foliage resulting in reduced leaf and corm yield (Levin, 2006; Martin, 2004).
3.2 Nematodes
The nematode Hirschmanniella miticausa causes the unique “miti miti” disease and was first reported out of the Solomon Islands in 1983 (Bridge, Mortimer, & Jackson, 1983). This species of nematode is also found in PNG. Hirschmanniella miticausa infestation results in dry brown rot of the corm, about 1-10 mm in size that originate from the base of the corm. It is nearly impossible to identify the rot symptoms until corms are harvested – chlorosis and wilting of leaves are indicators (Carmichael et al., 2008). Root knot nematodes (Meloidogyne spp.) and lesion nematodes (Pratylenchus coffeae); fairly widely distributed across the Pacific cause roots knots and root decay, stunting and death in taro respectively. Substantial corm yield losses have been reported (Arakaki, 1993; Sipes & Arakaki, 1997; Torigoe, Fukunaga, & Muta, 2002).
3.3 Fungal Diseases
There are numerous diseases produced by fungi important in taro production. One of the most common throughout the PICs is corm rot caused by the soil-borne fungus Athelia rilfsii. It is also the pathogen for a post-harvest pinkish rot on the corms. Brown leaf spot, also known as cladosporium leaf spot caused by Cladosporium colocasiae and Orange leaf spot caused by Neojohnstonia colocasiae are also widespread throughout the Pacific. Brown leaf spot is sometimes referred to as ghost spot due to the characteristic reddish-brown spots or blotches being less pronounced on one side of the leaf surface (Carmichael et al., 2008; Parris, 1941).
Spongy black rot, Lasiodiplodia theobromae (previously Botryodiplodia theobromae), has been reported to infect taro in Guam, PNG, Samoa and the Solomon Islands while White spot of taro, Leptosphaerulina trifolii is found in American Samoa, PNG, Samoa, Solomon Islands and Tuvalu. Both of these fungi have been detected on other hosts in majority of the countries in the region. Corm and leaf spot as a result of Marasmiellus stenophyllus infection has only been recorded in American Samoa, Tahiti, Wallis and Futuna and on a different host in Fiji. The “shot hole” fungi, Phoma spp. occurs throughout the Pacific while the leaf blotch fungus Pseudocercospora colocasiae is more restricted in distribution and seen only in American Samoa, Fiji, New Caledonia, Samoa, Solomon Islands, Tahiti and Vanuatu (Carmichael et al., 2008). Locally, phyllosticta leaf spot (Phyl1osticta colocasiophila) has long been recognized to affect dryland taro. Black rot (Ceratocystis fimbriata), Rhizopus rot (Rhizopus stolonifer) and fusarium dry rot (Fusarium solani or other Fusarium spp.) are taro pathogens as well (Ooka, 1990). Sclerotium or Southern Blight (Sclerotium rolfsii) has also been detected in Hawaiian taro (Parris, 1941).
3.4 Oomycete Diseases
The most important disease caused by these fungus-like eukaryotic microorganisms is taro leaf blight (TLB), Phytophthora colocasiae - first reported out of Java, Indonesia by Marian Raciborski in 1900. It is a highly destructive pathogen that primarily attacks the leaves; petioles and corms are also prone to infection (Brooks, 2008). Corm yield losses of 25-50 percent in the Pacific and 25-35 percent in the Philippines have been reported. Susceptible varieties in Hawaii have been noted to lose 95 percent of leaf yield once infected (Brooks, 2005; S. Nelson, Brooks, & Teves, 2011). TLB decimated Samoan and the American Samoan taro industries during an outbreak in 1993-1994. Within two years, Samoa’s annual USD10 million industry plummeted to just a little over USD60,000 (0.5% of average annual export value). American Samoa, which was producing close to 400,000 kg of taro before the epidemic managed only 5,000 kg in 1995 (Brooks, 2008; Singh et al., 2012). TLB is believed to have been first reported in Hawaii in 1920, two years after being discovered in Guam in 1918 (Hunter, Pouono, & Semisi, 1998). It is now spread throughout the Pacific and also found in Asia, East Asia, Africa, the Caribbean and the Americas (Nelson et al., 2011).
Another important oomycete disease is pythium rot, also known as corm soft rot caused by many species within the genus Pythium. This disease was first reported in Hawaii in 1902 by Sedgwick. It may start at the base or side of the corms then proliferate upwards infecting the entire tuber. Parris (1941) reported that the color of the rots vary in diseased corms; from whitish yellow to shades of grey or blue to dark purple. This rotting affects the aboveground appearance of the plant making it look stunted as it slowly dies away (Carmichael et al., 2008). Pythium aphanidermatum, P. graminicola and P. splendens have been reported in Palau, Samoa and Hawaii associated with yield losses of up to 80 percent. P. myriotylum has also been found in diseased corms in Solomon Islands, Samoa and Hawaii. P. irregulare has only been reported out of New Caledonia and P. carolinianum only out of Hawaii (Ooka, 1990).
3.5 Bacterial Diseases
Bacterial soft rot caused by Erwinia chrysanthemi has been recorded in the Solomon Islands (Carmichael et al., 2008). When infected, taro corms develop an aqueous soft decay whitish to dark blue in color. The rot is accompanied by a pungent odor. Bacterial leaf spot, the result of Xanthomonas campestris pv dieffenbachiae infection is also present in Hawaii, however, the disease is not significant (Ooka, 1990).
3.6 Viral Diseases
Several viruses are important pathogens of Colocasia taro. One of the most destructive viral diseases of taro in the Pacific is alomae. Gollifer & Brown(1972) were the first to describe this disease which is confined to the Solomon Islands and PNG. Plants affected by alomae develop a feathery mosaic symptom, emerging leaves are crinkled and fail to open properly; the plant overall is stunted with thickened, twisted dark green leaves. It is understood that co-infection by CBDV and TaBV causes this disease (James, Kenten, & Woods, 1973; Ooka, 1990) while TaVCV has also been suggested as a possible candidate (Carmichael et al., 2008). Substantial yield losses occur as a result of plant death (Gollifer et al., 1978). Of the two viruses initially associated with alomae, CBDV is transmitted by the delphacid Tarophagus proserpina and TaBV by the mealybugs Planococcus citri and Pseudococcus longispinus (Gollifer et al., 1977; Macanawai et al., 2005).
Somewhat similarly destructive is the Colocasia bobone disease virus (CBDV), a putative rhabdovirus found only in PNG and the Solomon Islands. It is relatively widespread and causes the bobone disease (when not involved with TaBV – in that case alomae results). Plants suffering from bobone display severe stunting, distorted, thickened and stiff leaves. Galls may also develop on the petioles and large leaf veins. CBDV is vectored by the taro planthopper, Tarophagus proserpina (Gollifer et al., 1978). Some researchers believe alomae and bobone can simultaneously be seen on taro plants and this condition is attributable to the Alomae-Babone virus complex (ABVC) (Ivancic et al., 1993). A less destructive yet most widespread virus of the taro family in the PICs is the Dasheen mosaic virus (DsMV). First described in 1970 by Zettler et al., infected plants display an array of mosaic patterns on the leaves with mild to moderate distortions. DsMV, member of the genus Potyvirus is a stylet-borne (non-persistent) virus transmitted by several species of aphids, namely, Myzus persicae, Aphis craccivora and A. gossypii (Ooka, 1990).
Taro bacilliform virus (TaBV), also known as the Taro badnavirus is common in many countries throughout the region. The virus was first described by James et al.(1973) and the full viral genome was sequenced three decades later (Yanget al. 2003). TaBV produces latent, erratic vein yellowing, often near the leaf margin. Leaf blades may noticeably bend backwards and sometimes creasing is apparent (Carmichael et al., 2008). Taro vein chlorosis virus (TaVCV) is another understudied taro viral disease of the Pacific. First described in 1999 by Pearson et al., it was fully characterized in 2005 by Revill et al. and gained formal recognition as a member of the genus Nucleorhabdvirus in the family Rhabdoviridae. It has so far been reported in 9 countries; namely the Federated States of Micronesia, Fiji, New Caledonia, Palau, PNG, Solomon Islands, Tuvalu, Vanuatu and most recently in the United States of America in the state of Hawaii.
Taro leaves infected by TaVCV show a characteristic feather-like chlorosis. This feathery yellowing broadens between adjacent veins as the leaf ages. The chlorosis coalesces into a network and in later stages may become necrotic leading to a “tattered appearance” of the leaf margins. These symptoms usually show up at maximum growth, seldom on young plants. The vein-chlorosis produced by TaVCV is more expressive than TaBV induced chlorosis. Taro plants do not experience stunting and/or gall formation as a result of TaVCV infection which is typical of CBDV, another rhabdovirus (Carmichael et al., 2008). A fifth taro virus, Taro reovirus (TaRV) has also been reported out of PNG, Solomon Islands and Vanuatu, however, it’s etiology and symptomatology are not well understood (Revill et al., 2005b). Recently, Groundnut bud necrosis virus, GBNV (genus Tospovirus, family Bunyaviridae) was found infecting taro in India. GBNV causes mosaic, chlorotic spotting and necrotic flecking on the leaves; the infected plant is overall stunted (Sivaprasad et al., 2011). This however, has been an isolated case and new reports of GBNV infection on taro have not been published from elsewhere.
4.0 Taro vein chlorosis virus (TaVCV) (Nucleorhabdovirus, family Rhabdoviridae, order Mononegavirales)
4.1 Rhabdoviridae family of viruses
Rhabdoviridae is a virus family within the order Mononegavirales which also contains the Bornaviridae, Filoviridae, Nyamiviridae and Paramyxoviridae families. There are eleven assigned genera within the Rhabdoviridae family. These include Cytorhabdovirus (9 species), Ephemerovirus (5 species), Lyssavirus (14 species), Norvirhabdovirus (4 species), Nucleorhabdovirus (10 species), Perhabdovirus (3 species), Sigmavirus (7 species), Sprivivirus (2 species), Tibrovirus (2 species), Tupavirus (2 species) and Vesiculovirus (9 species). This family of viruses also includes 4 species who have not been assigned a genus (“International Committee on Taxonomy of Viruses,” 2014).
Family Rhabdoviridae, Genus Cytorhabdovirus
Species:
Barley yellow striate mosaic virus
Broccoli necrotic yellows virus
Festuca leaf streak virus
*Lettuce necrotic yellows virus
Lettuce yellow mottle virus
Northern cereal mosaic virus
Sonchus virus
Strawberry crinkle virus
Wheat American striate mosaic virus
Genus Nucleorhabdovirus
Species:
Datura yellow vein virus
Eggplant mottled dwarf virus
Maize fine streak virus
Maize Iranian mosaic virus
Maize mosaic virus
*Potato yellow dwarf virus
Rice yellow stunt virus
Sonchus yellow net virus
Sowthistle yellow vein virus
Taro vein chlorosis virus
Tab. 1: Members of the plant infecting genera Cytorhabdovirus and Nucleorhabdovirus
*Indicates type member of its genus. Adopted from: ICTV, online
Species of only two of the eleven genera; Cytorhabdovirus and Nucleorhabdovirus infect plants (Walker et al., 2000) (Table 1). The two genera are differentiated based on their sites of replication and maturation. Nucleorhabdoviruses multiply in the nucleus of host cell then accumulate in the perinuclear space, whereas Cytorhabdoviruses characteristically replicate and accumulate in the cytoplasm (Jackson, Francki, & Zuidema, 1987). Species within each of these two genera are segregated depending on the hosts they infect and vector specificity. Molecular and serological assays and now more common genomic sequence data are used as additional tools for species demarcation (Jackson et al., 2005). The main distinguishing feature of the family is the enveloped virions with bacilliform or bullet-shaped morphology. The single stranded negative sense RNA are encapsulated in these particles, size estimates of which range from 45 to 100 nm in width and 130 to 350 nm in length (Brown, 1987).
Dichorhavirus, a third plant infecting genera of the Rhabdoviridae family has been proposed, however it is yet to receive formal recognition from ICTV. The major distinguishing features asserted for this “free-floating” Dichorhavirus genus versus Nucleorhabdoviruses and Cytorhabdoviruses are un-enveloped particles, bi-partite (segmented) genomes of its members and transmission by the Brevipalpus mites (family Tenuipalpidae). The Orchid fleck virus (OFV) is suggested as the type member of this tentative, unendorsed genus (Dietzgen et al., 2014).
All rhabdovirus genomes seemingly code for five major viral proteins flanked by the leader (l) and trailer (t) regions in the order 3’-l- N, P, M, G, L -t-5’ in negative polarity. The first ORF upstream of the 5’ end encodes for the large L protein; conserved domain for the RNA dependent RNA polymerase which is responsible for the replication of the viral RNA and transcription. Upstream of this, the G ORF encodes for the glycoprotein. This is a structural protein which forms the spike-like projections on the surface that aids virus attachment to the infected cells. From the 3’ end, the N ORF encodes for the nucleoprotein; major constituent of the viral nucleocapsid. Downstream to this is the P ORF coding for the phosphoprotein (also called the M1 protein) which is a component of the viral polymerase. Further downstream is the M ORF which codes for the matrix (or M2) protein. These form the inner lining of the virus envelope and play a role in virus budding. Plant rhabdoviruses that have been fully sequenced so far contain one to four additional ORFs. These extra genes mostly occur at position X between the P and M genes or alternatively at position Y between the G and L genes (Healy, Banyard, & Fooks, 2013; “International Committee on Taxonomy of Viruses,” 2014; Jackson et al., 1987; Redinbaugh & Hogenhout, 2005; Walker et al., 2015, 2000).
4.2 The genus Nucleorhabdovirus
Nucleorhabdovirus is one of two plant virus genera in the family Rhabdoviridae. This genus was previously classified as Plant Rhabdovirus group B. Species in this genus have bacilliform or bullet shaped enveloped, usually straight particles inside which the unsegmented ssRNA genome is packaged (Figure 1). Upon infection, the virions replicate and mature in the nucleus of the host plant cell. The Nucleorhabdovirus genome is characterized by monopartite, single-stranded, linear, negative sense RNA; 11 kb to 15 kb long. See Table 1 for members of this genus (Brunt et al., 1996).
Virions of this genus are regular in shape and size, 170-380 nm long and 55-100 nm in diameter. Prominent spikes or surface projections are strewed evenly over the exterior of the virion. When uncoiled, the nucleocapsids are filamentous, with obvious regular cross-banding and a distinct central canal. The virions are also symmetrically helical with pitch of 4.2 - 4.7 nm. Typical virion composition is 1 percent nucleic acid, 70 percent protein and 25 percent lipid (Adams & Antoniw, n.d.).
Some of the fully sequenced members of the genus include Sonchus yellow net virus (SYNV) (Choi, Scholthof, & Jackson, unpublished data), Rice yellow stunt virus (RYSV) (Huang et al., 2003), Maize mosaic virus (MMV) (Reed et al., 2005), Maize Fine Streak Virus (MFSV) (Tsai et al., 2005), Taro vein chlorosis virus (TaVCV) (Revill et al., 2005a) (Potato yellow dwarf virus (PYDV) (Bandyopadhyay et al., 2010), Rice transitory yellowing virus (RTYV) suggested to be synonymous with RYSV (Hiraguri et al., 2010), Datura yellow vein virus (DYVV) (Dietzgen, Innes, & Bejerman, 2015) and Eggplant mottled dwarf virus (EMDV) (G. Babaie et al., 2015). All have characteristic six or seven ORFs.
Fig. 1: putative structure of the members of the Nucleorhabdovirus genus
Adopted from: http://viralzone.expasy.org/all_by_species/78.html
4.3 TaVCV – known distribution and history
TaVCV is confirmed to occur in the Federated States of Micronesia, Fiji, New Caledonia, Palau, Papua New Guinea, Solomon Islands, Tuvalu and Vanuatu (Carmichael et al., 2008). The disease was first described and the name “Taro vein chlorosis virus” was first coined by Pearson et al. (1999) following comparative analysis against the Colocasia babone disease virus, another member of the rhabdovirus family. The distinction was based on recorded differences in particle size, disease symptomatology and serological reactivity.
A 2002 virus survey revealed that TaVCV was relatively widespread in Fiji (Harding, Williams, & Jackson, 2002). The findings of this survey were preliminary and based on symptoms alone; the major objective was to collect fresh leaf tissues from plants exhibiting TaVCV symptoms for virus purification and indexing. Revill et al. (2005a) were the first to characterize the TaVCV genome of a Fijian isolate and confirm the virus as a definitive member of the genus Nucleorhabdovirus. Their confirmation was based on the presence of six ORFs in the TaVCV anti-genome; equivalent to the N, P, 3, M, G and L genes common in the Rhabdoviridae family. This full genome sequence analysis was supplemented by thin-section electron microscopy data.
In addition to characterizing TaVCV, the group conducted a variability study and claimed high sequence diversity among Pacific strains of TaVCV. Isolates from Fiji, the Federated States of Micronesia, New Caledonia, Papua New Guinea, Solomon Islands and Vanuatu were used for comparison. Furthermore, their analysis showed that TaVCV is most closely related to Maize mosaic virus (MMV) – the reference strain of the Nucleorhabdovirus genus. The deduced amino acid sequences of the TaVCV ORFs had 63.1 (N), 46.1 (P), 43.4 (gene 3), 46.4 (M), 49.9 (G) and 67.9 (L) percent identity with the deduced amino acid sequences of the respective MMV ORFs. Finally, phylogenetic analysis utilizing the L gene deduced amino acid sequences grouped TaVCV in a clade with all other sequenced Nucleorhabdoviruses, separate from the Cytorhabdovirus clade. No further research on TaVCV has been published thereafter.
4.4 Symptomatology
An undated online fact sheet authored by Rob Harding adopted into a taro pest guide produced by the Australian Centre for International Agricultural Research in 2008 provide insights into the symptomatology and effects of the disease exerted on the plant by TaVCV. Infected leaves develop feather like, striding chlorosis of the veins that will often start near the leaf margin. This distinct vein chlorosis is more expressive than the vein chlorosis elicited by TaBV. The yellowing spreads between the veins and forms a network as the leaf ages. The chlorotic areas in certain cases become necrotic and the leaf margins consequently develop a ragged appearance (Figures 2a – d). There is no exact count on the number of leaves that may produce symptoms once a plant contracts the virus. Field observations indicate that new leaves which emerge after this infected series of leaves look healthy. Examination of leaves for typical symptoms can provide a basis for preliminary detection, however, symptoms may differ pertaining to cultivar and environmental conditions hence laboratory testing is required.
In comparison to symptoms produced by CBDV, which is also a rhabdovirus, TaVCV does not produce galls on the leaf blades and petioles nor causes stunting of the taro plants. Another unique feature of TaVCV in contrast to other taro virus diseases is that symptoms commonly occur when plants are at maximum growth and not at early stages of the life cycle (Carmichael et al., 2008; Harding, n.d.). TaVCV’s recent discovery coupled with the lack of research into the disease has left many uninvestigated parameters and is one of the reasons why the effect of this disease on corm yield is unknown.
Fig. 2a – d: characteristic symptoms of TaVCV on leaf samples collected from Big Island [Hawaii] (a), Oahu (b), Maui (c) and Kauai (d)
4.5 TaVCV description
4.5.1 Particle Morphology
Thin section electron microscopy analysis show that TaVCV virions measure approximately 200 x 70 nm in size (P. Revill et al., 2005) (Figure 3)
Fig. 3: Electron micrographs of cross-sections through a TaVCV-infected cell (a) showing the nucleus (N) containing TaVCV virions (V). Bar: 500 nm. The boxed area is enlarged in (b) and shows TaVCV virions (V) in the perinuclear space between the inner membrane (M) and outer membrane (E) of the nuclear envelope. Bar: 200 nm. Adopted from: Revill et al. (2005a)
4.5.2 Genome Organization
The negative sense ssRNA TaVCV genome is comprised of 12,020 nucleotides and contains six major ORFs. In agreement with other sequenced members of the Rhabdoviridae family, the TaVCV genome organization is 3’-leader-N-P-3-M-G-L-trailer-5’ (Figure 3). The length of these six ORFs are: 1506 (N), 813 (P), 861 (3), 705 (M), 1764 (G) and 5784 (L) nucleotides (Revill et al., 2005a). The function of gene 3 remains to be validated, although it was present at the same position as the sc4 gene of SYNV and gene 3 of MMV and RYSV. sc4 has been shown to be a putative movement protein (MP) in SYNV (Goodin et al. 2002; Scholthof et al. 1994). Likewise, gene 3 has a MP function in RYSV (Huang et al. 2005).
Fig. 3: Genome arrangement of TaVCV, in coding orientation. N, Putative nucleocapsid gene; P, putative phosphoprotein gene; 3, gene 3; M, putative matrix protein gene; G, glycoprotein gene; L, polymerase protein gene. Adopted from: Revill et al. (2005a)
4.5.3 Transmission
The vector for TaVCV is not known. The closely related CBDV, another member of the Rhabdoviridae family is vectored by the taro planthopper Tarophagus proserpina (family Delphacidae) (Gollifer et al., 1977). Other planthopper transmitted viruses of the genus Nucleorhabdovirus include MMV which are vectored by the corn planthopper Peregrinus maidis and Maize Iranian mosaic virus (MIMV) vectored by Ribautodelphax notabilis (ICTV, online). Leafhoppers (family Cicadellidae) are also prominent vectors of this genus. MFSV is transmitted by the blackfaced leafhopper Graminella nigrifrons (Redinbaugh et al., 2002; Todd et al. 2010). RYSV is transmitted by green rice leafhoppers Nephotettix nigropictus, N. cincticeps, and N. virescens (Chiu et al., 1965; Hiraguri et al., 2014). PYDV is transmitted by clover leafhoppers of the species Aceratagallia sanguinolenta (Black, 1943) whereas EMDV is vectored by the leafhoppers Anaceratogallia laevis, A. ribauti and Agallia vorobjevi (Babaie & Izadpanah, 2003); Sorghum stunt mosaic virus (SSMV) by Graminella sonora and Wheat American striate mosaic virus (WASMV) by Endria inimical and Graminella nigrifrons (ICTV, online). TaVCV is unlikely to be spread by seed or pollen. Conversely, it is certainly spread through the use of infected planting material (Harding, n.d.; Long et al., 2014; Revill et al., 2005b). Based on these relationships, presumably TaVCV is most likely vectored by a planthopper or leafhopper. Three species of Tarophagus have been described; T. colocasiae, T. persephone and T. proserpina with varying distribution around Asia and the Pacific (Asche & Wilson, 1989). However, any associations cannot be made with TaVCV transmission unless studies are able to provide evidence by employing molecular and serological techniques.
Materials and Methods
Objective 1: Determine the genetic diversity of TaVCV in Hawaii
Problem:
TaVCV, first reported in Hawaii in 2013, has not been thoroughly studied hence the genetic variability of the virus in Hawaii is unknown.
Approach:
Part 1: Sample Collection and Background
There are over 100 commercial taro farms across the state registered with the Hawaii Department of Agriculture. Taro from as many of these farms as possible will be surveyed by collecting approximately 100 cm2 of leaf tissue from plants with putative viral symptoms as well as from healthy controls. Approximately 10-20 plants from each site will be sampled. In addition, the University of Hawaii maintains a Hawaiian taro germplasm collection of approximately 75 varieties in four locations across the state. Each of these plants will also be sampled. All samples, including those displaying unusual symptoms will be documented, photographed and hyperlinked into a photo-database. All samples will be kept on ice during collection, and stored at -20 to -80oC in the laboratory until processed.
Part 2: Nucleic Acid Extraction (RNA), RT-PCR and Molecular Cloning
Total RNA will be isolated from approximately 100 mg of leaf tissue using a Macherey-Nagel NucleoSpin® RNA II kit (Thermo Fisher Scientific) following the manufacturers protocol. These RNAs will be reverse-transcribed into cDNA using the MMLV reverse transcriptase in a 20µl reaction. This cDNA will be used as template in PCR with virus-specific primers similar to the protocol employed by Revill et al. (2005a). However, in a 20 µl PCR reaction,10 µl 2 x GoTaq Green Master Mix (Promega Corporation) will be used instead of 1 x Titan reaction buffer, 1 mM dNTPs and 0.5 µl Titan polymerase mix; to this, 1 µl each of the 2 primers, 7 µl distilled water and 1 µl cDNA will be added. For PCR, the reactions will be subjected to a denaturing step at 95°C for 5 minutes and then 35 cycles of 95°C for 30 seconds, 55°C for 30 seconds and 72°C for 1 minute, with a final extension of 72°C for 5 minutes. Amplicons will be separated by electrophoresis through a 1% TAE/Agarose gel with Ethidium Bromide dye. TaVCV bands will be confirmed against a DNA ladder (Thermo Fisher Scientific) over UV light, photographed and printed for comparative analysis. Amplicons (1 µl) will then be ligated into 0.5 µl pGEM-T vector in a 10 µl reaction using 5 µl 2 x Ligation buffer, 1 µl T4 DNA Ligase and 2.5 µl distilled water. The plasmids will be incorporated into E. coli DH5α cells and incubated in SOB medium for 45 minutes, then plated on McConkey agar for transformed colony selection. Selected colonies will be cultured in LB broth overnight then purified using the Mini-Prep Lab – Qiagen QIA prep spin protocol and sequenced at the Advanced Studies in Genomics, Proteomics and Bioinformatics (ASGPB) Laboratory or Greenwood Biotech Core Laboratory using 3.2 pmol SP6 and T7 primers in a 8 µl sequence reaction.
Part 3: Sequence and Phylogenetic Analysis
Sequence results would be compared with databases in the National Center for Biotechnology Information (NCBI) website. Each sequence would be confirmed in at least three clones (to get a consensus of three sequences) and a contiguous sequence would be generated using the Cap 3 Sequence Assembly Program or similar software. The consensus nucleotide sequences will be used to generate protein sequences at the EXPASY website (www.expasy.org).
Both the nucleotide and deduced amino acid sequences will be aligned using the ClustalX program and neighbor-joined phylogenetic trees will be constructed using bioinformatics softwares such as Geneious. Both nucleotide and protein sequences will also be subjected to Maximum Likelihood analysis. Character Algorithms will also be studied using the Bayesian (MRBAYES) method to obtain an understanding of the diversity of TaVCV strains in Hawaii.
Objective 2: Develop reliable molecular and serological diagnostic tests to detect variant TaVCV isolates in Hawaii
Problem:
Study of TaVCV isolates from six Pacific Island countries, namely Fiji, Federated States of Micronesia, New Caledonia, Papua New Guinea, Solomon Islands and Vanuatu identified high levels of sequence diversity amongst those strains (Revill et al., 2005a). In view of the aforementioned, the possibility that current PCR based assays may not detect all isolates is high. Therefore, development of a robust detection assay is required.
Approach:
Part 1: Design better RT-PCR Primers
The research will identify conserved regions in the virus sequences obtained in Objective 1 to design better degenerate and specific primers using various online primer design softwares to improve the RT-PCR assay that will detect diverse TaVCV strains. A protocol slightly modified from McGavin, Cock, & MacFarlane (2011) will be employed.
Part 2: Antibody Production
Technique 1: The nucleocapsid (N) gene will be sequenced from various isolates. Nucleotide sequences will then translated into protein sequences using the EXPASY website then outsourced for antibody production. Probable companies that maybe used for designing antibodies include Thermo Fisher Scientific – Pierce Antibody Products, Biomatik, Rockland Immunochemicals, Genscript and Pacific Immunology.
Technique 2: TaVCV will be purified from infected taro plants by Celite filtration and sucrose density gradient centrifugation; using a modification of the procedure described by Creamer (1992). The purified virus will then be used as antigen for mono or polyclonal antibody production.
Part 3: Evaluation
The antibodies obtained from either of the above two techniques will then be tested against the local strains of TaVCV in an Enzyme-Linked Immunosorbent Assay (ELISA) to develop a serological detection method for TaVCV. This ELISA technique (or the antibodies) will be shared with other researchers to test the effectiveness and efficiency of the assay.
Objective 3: Identify the vector of TaVCV
Problem:
Despite its recognition as an important viral leaf disease of taro, the vector(s) which naturally transmit the virus in the field is/are unknown.
Approach:
TaVCV infected taro plants, in other words, the donor or source plants will be grown in a nursery or lab under optimum conditions. Suspected vector insects (Delphacids [plant hoppers], mealybugs and aphids– listed here in order of priority) will be reared and allowed to feed on these plants. Appropriate number of insects (identified by preliminary RT-PCR tests) will be transferred to a single uninfected plant (called the recipient or test plant); preferably 5 – 10 plants for replication. As negative control, TaVCV uninfected taro plants will also be used as donor or source plants. Visual disease symptoms will be scored for TaVCV after suspected vector introduction onto test plants and confirmed by PCR/ELISA.
Literature Cited
Abbas. (2011). Taro/ Taro Root - Health Benefit and Uses: Taro and Beef Curry Recipe. Retrieved October 14, 2014, from http://herbs-treatandtaste.blogspot.com/2011/07/taro-taro-root-health-benefits-and-uses.html
Adams, M. J., & Antoniw, J. F. (n.d.). Notes on Genus: Nucleorhabdovirus. Retrieved September 29, 2015, from http://www.dpvweb.net/notes/showgenus.php?genus=Nucleorhabdovirus
Agrios, G. N. (2005). Plant Pathology. (K. D. Sonnack, Ed.) (Fifth). 30 Corporate Drive, Suite 400, Burlington, MA 01803, USA: Elsevier Academic Press.
Alcantara, R. M., Hurtada, W. A., & Dizon, E. I. (2013). The Nutritional Value and Phytochemical Components of Taro [Colocasia esculenta (L.) Schott] Powder and its Selected Processed Foods. Journal of Nutrition & Food Science, 3(3), 1–7.
Alercia, A. (2013). Nutritious Underutilized Species - Taro (Colocasia esculenta L.). Rome: Bioversity International, Via dei Tre Denari 472/a, 00057 Maccarese (Fiumicino). Retrieved from http://www.bioversityinternational.org/e-library/publications/detail/nutritious-underutilized-species-taro/
Aloalii, I., Masamdu, R., Theunis, W., & Thistleton, B. (1993). Prospects for Biological Control of Taro Beetles, Papuana spp. (coleoptera: scarabaeidae), in the South Pacific. Research Extension Series: Hawaii Institute of Tropical Agriculture and Human Resources, 66–70.
Ammar, M. S., Hegazy, A. E., & Bedeir, S. H. (2009). Using of Taro Flour as Partial Substitute of Wheat Flour in Bread Making. World Journal of Dairy & Food Sciences, 4(2), 94–99.
Arakaki, A. (1993). Relationship between Nematode Populations and Corm Rot in “Bun Long” Taro Production. Research Extension Series - Hawaii Institute of Tropical Agriculture and Human Resources, 71–72.
Asche, M., Wilson, M. R. (1989). The three taro planthoppers: species recognition in Tarophagus (Hemiptera: Delphacidae). Bulletin of Entomological Research, 79(2), 285–298. doi:10.1017/S0007485300018277
Babaie, G. H., & Izadpanah, K. (2003). Vector Transmission of Eggplant Mottled Dwarf Virus in Iran. Journal of Phytopathology, 151, 679–682.
Babaie, G., Habibi, M. K., Massah, A., Dizadji, A., Izadinejad, L., & Simon, A. (2015). Complete Genome Sequence and Genome Analysis of Eggplant mottled dwarf virus-Iranian Isolate. Journal of Phytopathology , 163(5), 331–341.
Bandyopadhyay, A., Kopperud, K., Anderson, G., Martin, K., & Goodin, M. (2010). An integrated protein localization and interaction map for Potato yellow dwarf virus, type species of the genus Nucleorhabdovirus. Virology, 402(1), 61–71. doi:10.1016/j.virol.2010.03.013
Bishop, R. V. (2013, December). The mother of our breath. Retrieved October 14, 2014, from http://www.agriculturesnetwork.org/magazines/global/family-farming/palau-family-values
Black, L. M. (1943). Genetic Variation in the Clover Leafhopper’s ability to transmit Potato Yellow Dwarf Virus. Genetics, 28, 200–209.
Bridge, J., Mortimer, J. J., & Jackson, G. V. H. (1983). Hirschnzanniella miticausa n. sp. (Nematoda : Pratylenchidae) and its pathogenicity on taro (Colocasia esculenta). Revue Nematol, 6(2), 285–290.
Brooks, F. E. (2005). Taro leaf blight. doi:DOI:10.1094/PHI-I-2005-0531-01
Brooks, F. E. (2008). Detached-leaf bioassay for evaluating taro resistance to Phytophthora colocasiae. Plant Disease, 92, 126–131.
Brown, A. C., & Valiere, A. (2004). The Medicinal Uses of Poi. Nutrition in Clinical Care, 7(2), 69–74.
Brown, F. (1987). The Family Rhabdoviridae - General Description and Taxonomy. In R. R. Wagner (Ed.), The Rhabdoviruses, Part of the series The Viruses (pp. 1–8). New York: Plenum.
Brunt, A. A., Crabtree, K., Dallwitz, M. J., Gibbs, A. J., Watson, L., & Zurcher, E. J. (eds. . (1996). Plant Viruses Online: Descriptions and Lists from the VIDE Database. Version: 20th. Retrieved October 20, 2015, from http://biology.anu.edu.au/Groups/MES/vide/
Caillon, S., Garcia, J. Q., & Guarino, L. (2004, March). Taro in Vanuatu: towards a dynamic conservation strategy. LEISA Magazine, 18–20. Retrieved from http://www.agriculturesnetwork.org/magazines/global/valuing-crop-diversity/taro-in-vanuatu-towards-a-dynamic-conservation
Carmichael, A., Harding, R., Jackson, G., Kumar, S., Lal, S. N., Masamdu, R., … Clarke, A. R. (2008). Taro Pest - An illustrated guide to pests and diseases of taro in the South Pacific. ACIAR MONOGRAPH SERIES. Canberra: Australian Centre for International Agricultural Research (ACIAR).
Chiu, R. J., Lo, T. C., Pi, C. L., & Chen, M. H. (1965). Transitory yellowing of Rice and its transmission by the leaf hopper Nephotettix apicalis apicalis (Motsch). Botanical Bulletin of Academia Sinica, 6(1), 1–18.
Cho, J. J., Yamakawa, R. A., & Hollyer, J. (2007, February). Hawaiian Kalo, Past and Future. Sustainable Agriculture. Honolulu: University of Hawaii - College of Tropical Agriculture and Human Resources. Retrieved from http://www.ctahr.hawaii.edu/oc/freepubs/pdf/SA-1.pdf
Choi, T.-J., Scholthof, K.-B. G., & Jackson, A. O. (n.d.). Complete nucleotide sequence determination of Sonchus yellow net virus, a plant rhabdovirus. Retrieved from http://www.ncbi.nlm.nih.gov/nuccore/L32603.1
Creamer, R. (1992). Purification and Protein Characterization of Sorghum Stunt Mosaic Rhabdovirus. Phytopathology, 82, 1473–1476.
CTAHR and Taro. (2009). Honolulu: College of Tropical Agriculture and Human Resources. Retrieved from http://www.ctahr.hawaii.edu/oc/freepubs/pdf/CTAHR_and_taro.pdf
Davis, R. I., & Ruabete, T. K. (2010). Records of plant pathogenic viruses and virus-like agents from 22 Pacific island countries and territories: a review and an update. Australasian Plant Pathology, 39(3), 265–291. doi:10.1071/AP10047
Deo, P. C., Tyagi, A. P., Taylor, M., Becker, D. K., & Harding, R. M. (2009). Improving taro (Colocasia esculenta var. esculenta) production using biotechnological approaches. South Pacific Journal of Natural Science, (27), 6–13. Retrieved from http://eprints.qut.edu.au/29273/1/29273.pdf
Dietzgen, R. G., Innes, D. J., & Bejerman, N. (2015). Complete genome sequence and intracellular protein localization of Datura yellow vein nucleorhabdovirus. Virus Research, 205, 7–11.
Dietzgen, R. G., Kuhn, J. H., Clawson, A. N., Freitas-Astúa, J., Goodin, M. M., Kitajima, E. W., … Whitfield, A. E. (2014). Dichorhavirus: A proposed new genus for Brevipalpus mite-transmitted, nuclear, bacilliform, bipartite, negative-strand RNA plant viruses. Archives of Virology, 159(3), 607–619. doi:10.1007/s00705-013-1834-0
Ellis, S. D., Boehm, M. J., & Feng, Q. (2008). Viral Diseases of Plants. Agriculture and Natural Resources Factsheet. Ohio: Ohio State University. Retrieved from http://ohioline.osu.edu/hyg-fact/3000/pdf/PP401_05.pdf
Gollifer, D. E., & Brown, J. F. (1972). Virus diseases of Colacasia esculenta in the British Solomon Islands. Plant Disease Reporter, 56(7), 597–599.
Gollifer, D. E., Jackson, G. V. H., Dabek, A. J. and, & Plumb, R. T. (1978). Incidence, and effects on yield, of virus diseases of taro (Colocasia esculenta) in the Solomon Islands. Annals of Applied Biology, 88(1), 131–135. doi:10.1111/j.1744-7348.1978.tb00687.x
Gollifer, D. E., Jackson, G. V. H., Dabek, A. J., Plumb, R. T., & May, Y. Y. (1977). The Occurrence and Transmission of Viruses of Edible Aroids in the Solomon Islands and the Southwest Pacific. PANS, 23(2), 171–177. doi:10.1080/09670877709412425
Gomes, A. (2012, December). Revenue from crops surges 7% to top $700M. Star Advertiser. Honululu. Retrieved from http://www.staradvertiser.com/business/20121223_Revenue__from_crops_surges_7__to_top_700M.html?id=184585611
Goodin, M. M., Dietzgen, R. G., Schichnes, D., Ruzin, S., & Jackson, A. O. (2002). pGD vectors: versatile tools for the expression of green and red fluorescent protein fusions in agroinfiltrated plant leaves. The Plant Journal, 31(3), 375–383.
Greenwell, A. B. H. (1947). Taro: With Special Reference to Its Culture and Uses in Hawaii. Economic Botany, 1(3), 276–289.
Harding, R. (n.d.). Taro vein chlorosis virus Fact Sheet. Brisbane: Queensland University of Technology. Retrieved from http://www.ediblearoids.org/portals/0/taropest/lucidkey/taropest/media/Html/Viruses/TaroVCV/TaroVCV.htm
Harding, R. (2008). Viruses of Taro in the Pacific. Agriculture for Development, 2, 21–25. Retrieved from http://www.taa.org.uk
Harding, R., Williams, B., & Jackson, G. (2002). Taro Virus Survey of the South Pacific Fiji. Retrieved from http://www.ediblearoids.org/Portals/0/Documents/WP7Viruses/Fiji taro virus survey.pdf
Healy, D. M., Banyard, A. C., & Fooks, A. R. (2013). Rhabdoviruses. In eLS. Chichester: John Wiley & Sons Ltd. doi:10.1002/9780470015902.a0001085.pub3
Hill, A. F. (1939). The Nomenclature of the Taro and its Varieties. Botanical Museum Leaflets. Harvard University, 7(7), 113 – 118.
Hiraguri, A., Hibino, H., Hayashi, T., Shimizu, T., Uehara-Ichiki, T., Omura, T., & Sasaya, T. (2010). Complete sequence analysis of rice transitory yellowing virus and its comparison to rice yellow stunt virus. Archives of Virology, 155(2), 243–245.
Hiraguri, A., Netsu, O., Sasaki, N., Nyunoya, H., & Sasaya, T. (2014). Recent progress in research on cell-to-cell movement of rice viruses. Frontiers in Microbiology, 5, 1–10. doi:10.3389/fmicb.2014.00210
Hollyer, J., Paull, R., & Huang, A. (2000). Processing Taro Chips. Food Manufacturing and Technology, 1, 1–2.
Hu, J. S., Meleisea, S., Wang, A., Shaarawy, M. A., & Zettler, F. W. (1995). Dasheen mosaic potyvirus in Hawaiian taro. Australasian Plant Pathology, 24(2), 112–117.
Huang, Y. W., Geng, Y. F., Ying, X. B., Ying, X., & Fang, R. X. (2005). Identification of a Movement Protein of Rice Yellow Stunt Rhabdovirus. Journal of Virology, 79(4), 2108–2114. doi:10.1128/JVI.79.4.2108-2114.2005
Huang, Y., Zhao, H., Luo, Z., Chen, X., & Fang, R. X. (2003). Novel structure of the genome of Rice yellow stunt virus: identification of the gene 6-encoded virion protein. J. Gen. Virol., 84(8), 2259–2264.
Hunter, D., Pouono, K., & Semisi, S. (1998). The Impact of Taro Leaf Blight in the Pacific Islands with special reference to Samoa. J. S. Pac. Agr. , 5, 44–56.
International Committee on Taxonomy of Viruses. (2014). doi:http://www.ictvonline.org/index.asp
Ivancic, A., Liwqula, R., Levela, H., Saelea, J., & Wagatora, D. (1993). Genetic Resistance to Alomae-Bobone Virus Complex The Lethal Disease of Taro (Colocasia esculenta (L.) Schott). Honolulu.
Jackson A. O., Dietzgen R. G.’, Goodin M. M., Bragg J. N., & Deng M. (2005). Biology of Plant Rhabdoviruses. Annual Review of Phytopathology, 43, 623–660. doi:10.1146/annurev.phyto.43.011205.141136
Jackson, A. O., Francki, R. I. B., & Zuidema, D. (1987). Biology, structure and replication of plant rhabdoviruses. In The Rhabdoviruses. In R. R. Wagner (Ed.), The Rhabdoviruses, Part of the series The Viruses (pp. 427–508). New York: Plenum.
Jacobs, R. (2011). Kalo Is More Than a Native Hawaiian Plant—It’s an Ancestor to Hawaiian Culture. Retrieved from http://indiancountrytodaymedianetwork.com/2011/11/21/kalo-more-native-hawaiian-plant-its-ancestor-hawaiian-culture-63402
James, M., Kenten, R. H., & Woods, R. D. (1973). Virus-like particles associated with two diseases of Colocasia esculenta (L.) Schott in the British Solomon Islands. Journal of General Virology, 21, 145–153.
Jane, J., Shen, L., Chen, J., Lim, S., Kasemsuwan, T., & Nip, W. K. (1992). Physical and Chemical Studies of Taro Starches and Flours. Cereal Chemistry, 69(5), 528–535.
Korotieieva, H. V., & Polishchuk, V. P. (2004). Viruses of orchids of the natural flora in Ukraine. Mikrobiol Z., 66(2), 74–80.
Kreike, C. M., Van Eck, H. J., & Lebot, V. (2004). Genetic diversity of taro, Colocasia esculenta (L.) Schott, in Southeast Asia and the Pacific. TAG. Theoretical and Applied Genetics. Theoretische Und Angewandte Genetik, 109(4), 761–768. doi:10.1007/s00122-004-1691-z
Kuruvilla, K. M., & Singh, A. (1981). Karyotypic and electrophoretic studies on taro and its origin. Euphytica, 30(2), 405–413.
Lal, S., Moxon, J., Autrar, M., Milner, R. J., Hunter, D., Hazelman, S., … Vasuidreketi, T. (2008). Taro Beetle Management in Papua New guinea and Fiji: Final Project Report. Suva. doi:978-982-00-0304-0
Lebot, V., & Aradhya, K. M. (1991). Isozyme variation in taro (Colocasia esculenta (L.) Schott) from Asia and Oceania. Euphytica, 56(1), 55–66.
Lee, W. (1999). Taro (Colocasia esculenta). Retrieved from http://www.ethnoleaflets.com/leaflets/taro.htm
Levin, P. (2006). Statewide Strategic Control Plan for Apple Snail (Pomacea canaliculata) in Hawai’i. Honolulu.
Liloqula, R., Saelea, J., & Levela, H. (1993). Traditional Taro Cultivation in the Solomon Islands. In L. Ferentinos (Ed.), Sustainable Taro Culture for the
Pacific Conference; 1992, September 24-25 (pp. 125–131). Honolulu: University of Hawaii.
Long, M. H., Ayin, C., Li, R., Hu, J. S., & Melzer, M. J. (2014). First Report of Taro Vein Chlorosis Virus Infecting Taro (Colocasia esculenta) in the United States. Plant Disease, 98(8), 1160–1160. doi:10.1094/PDIS-12-13-1277-PDN
Macanawai, A. R., Ebenebe, A. A., Hunter, D., Devitt, L. C., Hafner, G. J., & Harding, R. M. (2005). Investigations into the seed and mealybug transmission of Taro bacilliform virus. Australasian Plant Pathology, 34(1), 73–76. doi:10.1071/AP04084
Martin, D. A. (2004). Hawaii Taro. Honolulu. Retrieved from http://www.nass.usda.gov/Statistics_by_State/Hawaii/Publications/Archive/xtar03.pdf
Matthews, P. J. (1995). Aroids and the Austronesians. Tropics, 4(2), 105–126. doi:10.3759/tropics.4.105
Matthews, P. J. (2004). Genetic Diversity in Taro, and the Preservation of Culinary Knowledge. Ethnobotany Research and Applications, 2, 55–71.
McGavin, W. J., Cock, P. J. A., & MacFarlane, S. A. (2011). Partial sequence and RT-PCR diagnostic test for the plant rhabdovirus Raspberry vein chlorosis virus. Plant Pathology, 60(3), 462–467. doi:10.1111/j.1365-3059.2010.02387.x
McGregor, A., Afeaki, P., Hamilton, A., Hollyer, J., Masamdu, R., & Nalder, K. (2011). Pacific Island Taro Market Access Scoping Study. Suva, Fiji.
Melzer, M., Nelson, S., & Borth, W. (2013). Hawaii Plant Disease. Retrieved from http://hawaiiplantdisease.net/Plant-virus-diseases-in-Hawaii.php
Moore, L. M., & Lawrence, J. H. (2003). Plant Guide - Taro. United States Department of Agriculture - Natural Resources Conservation Service. Retrieved from http://plants.usda.gov/plantguide/pdf/cs_coes.pdf
Nelson, S., Brooks, F., & Teves, G. (2011). Taro Leaf Blight in Hawai‘i. Plant Disease, 71, 1–14.
Nelson, S. C. (2008). Dasheen Mosaic of Edible and Ornamental Aroids. Plant Disease, 44, 1–9.
Noyer, J. L., Billot, C., Weber, A., Brottier, P., Quero-Garcia, J., & Lebot, V. (2003). Genetic diversity of Taro (Colocasia esculenta (L.) Schott) assessed by SSR markers. In Third Taro Symposium, SPC-TPGRI-FAO-CIRAD (pp. 1–9). Nadi, Fiji.
Onwueme, I. (1999). Taro Cultivaion in Asia and the Pacific. Bangkok, Thailand: Food and Agriculture Organization of the United Nations, Regional Office for Asia and the Pacific.
Ooka, J. J. (1990). Taro Diseases. Research Extension series - Hawaii Institute of Tropical Agriculture and Human Resources. Honolulu. Retrieved from http://www.ctahr.hawaii.edu/oc/freepubs/pdf/RES-114-11.pdf
Parris, G. K. (1941). DISEASES OF TARO IN HAWAII AND THEIR CONTROL - With Notes on Field Production. Hawaii Agricultural Experiment Station of the University of Hawaii Circular, 18, 9–28. Retrieved from http://www.ctahr.hawaii.edu/oc/freepubs/pdf/C2-18.pdf
Payne, J. H., Ley, G. J., & Akau, G. (1941). Processing and Chemical Investigations of Taro. Honolulu: Hawaii Agricultural Experiment Station - University of Hawaii.
Pearson, M. ., Jackson, G. V. H., Saelea, J., & Morar, S. G. (1999). Evidence for two rhabdoviruses in taro (Colocasia escudenta) in the Pacific region. Australasian Plant Pathology, 28(3), 248–253. doi:10.1071/AP99040
Pests in Brief. (2013). Pacific Pest Detector News Number 15, September 2013 - A Quarterly Newsletter for First Detectors. Retrieved from https://www.wpdn.org/webfm_send/489
Plucknett, D. L. (1983). Taxonomy of the Genus Colocasia. In J.-K. Wang & S. Higa (Eds.), Taro: A Review of Colocasia esculenta and its Potentials (pp. 14–19). Honolulu: University of Hawaii Press.
Pole, F. S. (1993). Continuing Role of Aroids in the Root Crop-Based Cropping System of Tonga. In L. Ferentinos (Ed.), Sustainable Taro Culture for the Pacific Conference; 1992, September 24-25 (pp. 11–14). Honolulu: University of Hawaii.
Potgieter, M. (1940). Taro (Colocasia esculenta) as a food. Journal of the American Dietetic Association, 16, 536–540.
Primo, A. (1993). Colocasia Taro on Pohnpei Island. In L. Ferentinos (Ed.), Sustainable Taro Culture for the Pacific Conference; 1992, September 24-25 (pp. 6–7). Honolulu: University of Hawaii.
Purseglove, J. W. (1973). Tropical Crops: Monocotyledons Vols. 1 and 2 . Experimental Agriculture, 9(3), 287.
Rao, V. R., Hunter, D., Eyzaguirre, P. B., & Matthews, P. J. (2010). Ethnobotany and global diversity of taro. In V. R. Rao, P. J. Matthews, P. B. Eyzaguirre, & D. Hunter (Eds.), The Global Diversity of Taro Ethnobotany and Conservation (pp. 1–6). Rome: Bioversity International.
Redinbaugh, M. G., & Hogenhout, S. A. (2005). Plant rhabdoviruses. Curr Top Microbiol Immunol., 292, 143–163.
Redinbaugh, M. G., Seifers, D. L., Meulia, T., Abt, J. J., Anderson, R. J., Styer, W. E., … Hogenhout, S. A. (2002). Maize fine streak virus, a New
Leafhopper-Transmitted Rhabdovirus. Phytopathology, 92, 1167–1174.
Reed S. E., Tsai C. W., Willie, K. J., Redinbaugh, M. G., & Hogenhout, S. A. (2005). Shotgun sequencing of the negative-sense RNA genome of the rhabdovirus Maize mosaic virus. J Virol Methods, 129(1), 91–96.
Revill, P. A., Jackson, G. V. H., Hafner, G. J., Yang, I., Maino, M. K., Dowling, M. L., … Harding, R. M. (2005). Incidence and distribution of viruses of Taro (Colocasia esculenta) in Pacific Island countries. Australasian Plant Pathology, 34(3), 327–331. doi:10.1071/AP05032
Revill, P., Trinh, X., Dale, J., & Harding, R. (2005). Taro vein chlorosis virus: characterization and variability of a new nucleorhabdovirus. Journal of General Virology, (86), 491–499. doi:10.1099/vir.0.80591-0
Rodoni, B. C., Dale, J. L., & Harding, R. M. (1994). Review of alomae disease of taro. Papua New Guinea Journal of Agriculture, Forestry and Fisheries, 37(2), 14–18.
Sami, R. (2011). What’s holding Pacific Island taro exports back? Retrieved October 14, 2014, from http://www.spc.int/lrd/fact/press-releases/whats-holding-pacific-island-taro-exports-back
Sato, D. M., Hara, A. H., Mau, R. F. L., Tsuda, D. M., & Hamasaki, T. (1997). Taro Root Aphid. Insect Pests, 1–2.
Scholthof, K. B., Hillman, B. I., Modrell, B., Heaton, L. A., & Jackson, A. O. (1994). Characterization and detection of sc4: a sixth gene encoded by sonchus yellow net virus. Virology, 204(1), 279–288.
Sedgwick, T. F. (1902). The Root Rot of Taro. Bulletin (Vol. 2). Honolulu.
Singh, D., Jackson, G., Hunter, D., Fullerton, R., Lebot, V., Taylor, M., … Tyson, J. (2012). Taro Leaf Blight—A Threat to Food Security. Agriculture, 2, 182–203. doi:10.3390/agriculture2030182
Sipes, B. S., & Arakaki, A. S. (1997). Root-knot Nematode Management in Dryland Taro with Tropical Cover Crops. Journal of Nematology, 29(4S), 721–724.
Sivan, P., & Liyanage, A. de S. (1993). Breeding and Evaluation of Taro (Colocasia esculenta) for the South Pacific Region. In L. Ferentinos (Ed.), Sustainable Taro Culture for the Pacific Conference; 1992, September 24-25 (pp. 1–5). Honolulu: University of Hawaii.
Sivaprasad, Y., Bhaskara Reddy, B. V., Naresh Kumar, C. V. M., Raja Reddy, K., & Sai Gopal, D. V. R. (2011). First report of Groundnut Bud Necrosis Virus infecting Taro (Colocasia esculenta). Australasian Plant Disease Notes, 6(1), 30–32.
Taotua, F. (1993). Taro Cultivatars in Samoa. In L. Ferentinos (Ed.), Sustainable Taro Culture for the Pacific Conference; 1992, September 24-25 (pp. 101–102). Honolulu: University of Hawaii.
Taro. (2014). Retrieved September 29, 2014, from http://www.hawaiihistory.org/index.cfm?fuseaction=ig.page&PageID=533
Taro Research. (2010). Retrieved September 28, 2014, from http://www.spc.int/lrd/research/taro-research
Temesgen, M., & Retta, N. (2015). Nutritional Potential, Health and Food Security Benefits of Taro Colocasia Esculenta (L.): A Review. Food Science and Quality Management, 36, 23–30.
Todd, J. C., Ammar, E. D., Redinbaugh, M. G., Hoy, C., & Hogenhout, S. A. (2010). Plant Host Range and Leafhopper Transmission of Maize fine streak virus. Phytopathology, 100, 1138–1145.
Torigoe, H., Fukunaga, M., & Muta, T. (2002). Control of stunting and wilting of taro, Colocasia esculenta, 3: Effect of organic matter on the root-lesion nematode, Pratylenchus coffeae, on tar. Retrieved October 31, 2015, from http://agris.fao.org/agris-search/search.do?recordID=JP2003000414
Trujillo, E. E., Menezes, T. D., Cavaletto, C. G., Shimabuku, R., & Fukuda, S. K. (2002). Promising New Taro Cultivars with Resistance to Taro Leaf Blight: ‘Pa“lehua”, ‘Pa“akala”, and “Pauakea.” New Plants for Hawaii, 7, 1–4.
Tsai, C. W., Redinbaugh, M. G., Willie, K. J., Reed, S., Goodin, M., & Hogenhout, S. A. (2005). Complete Genome Sequence and In Planta Subcellular Localization of Maize Fine Streak Virus Proteins. Journal of Virology, 79(9), 5304–5314. doi:10.1128/JVI.79.9.5304–5314.2005
Tuia, V. S., Wainaqabete, L. M., Sukal, A., Baculacula, S., Lutu, U., Shandil, A., … Prasad, R. (2012). Role of the Centre for Pacific Crops and Trees in the sustainable conservation and the safe movement of plant material. Suva: Centre for Pacific Crops and Trees, Secretariat of the Pacific Community.
Uchida, J., Levin, P., Miyasaka, S., Teves, G., Hollyer, J., Nelson, S., & Ooka, J. (2008). Taro Mauka to Makai. A Taro Production and Business Guide for Hawaii Growers. (D. Evans, Ed.) (Second). College of Tropical Agriculture and Human Resources, University of Hawaii.
USDA Crop Production 2013 Summary. (2014). doi:1057-7823
USDA Crop Values 2013 Summary. (2014). doi:1949-0372
Vilsoni, F. (1993). Sustainable Taro Culture: Fiji Situation. In L. Ferentinos (Ed.), Sustainable Taro Culture for the Pacific Conference; 1992, September 24-25 (pp. 84–87). Honolulu: University of Hawaii.
Walker, P. J., Benmansour, A., Dietzgen, R., & et al. (2000). Family Rhabdoviridae. In Virus Taxonomy and Nomenclature of Viruses. Seventh Report of the International Committee on Taxonomy of Viruses. San Diego.
Walker, P. J., Firth, C., Widen, S. G., Blasdell, K. R., Guzman, H., Wood, T. G., & et al. (2015). Evolution of Genome Size and Complexity in the Rhabdoviridae. PLoS Pathog , 11(2), 1–25. doi:10.1371/journal.ppat.1004664
Wang, J.-K., & Higa, S. (1983). Taro: A Review of Colocasia esculenta and its Potentials. Honolulu: University of Hawaii Press.
Whitney, L. D., Bowers, F. A. I., & Takahashi, M. (1939). Taro Varieties in Hawaii. Hawaii Agricultural Experiment Station of the University of Hawaii (Vol. 84). Honolulu.
Yalu, A., Singh, D., & Yadav, S. S. (2009). Taro Improvement and Development in Papua New Guinea - A Success Story. Bangkok, Thailand. Retrieved
from http://www.apaari.org/wp-content/uploads/2009/08/taro-papua-guinea-final.pdf
Yang, I. C., Hafner, G. J., Dale, J. L., & Harding, R. M. (2003). Genomic characterisation of taro bacilliform virus. Archives of Virology, 148(5), 937–49.
Zettler, F. W., Foxe, M. J., Hartman, R. D., Edwardson, J. R., & Christie, R. G. (1970). Filamentous viruses infecting Dasheen and other Araceous plants. Phytopathology , 60(6), 983–987. doi:10.1094/Phyto-60-983